Reference management. Clean and simple.

The top list of academic research databases

2. Web of Science
5. ieee xplore, 6. sciencedirect, 7. directory of open access journals (doaj), get the most out of your academic research database, frequently asked questions about academic research databases, related articles.
Whether you are writing a thesis , dissertation, or research paper it is a key task to survey prior literature and research findings. More likely than not, you will be looking for trusted resources, most likely peer-reviewed research articles.
Academic research databases make it easy to locate the literature you are looking for. We have compiled the top list of trusted academic resources to help you get started with your research:
Scopus is one of the two big commercial, bibliographic databases that cover scholarly literature from almost any discipline. Besides searching for research articles, Scopus also provides academic journal rankings, author profiles, and an h-index calculator .
- Coverage: 90.6 million core records
- References: N/A
- Discipline: Multidisciplinary
- Access options: Limited free preview, full access by institutional subscription only
- Provider: Elsevier
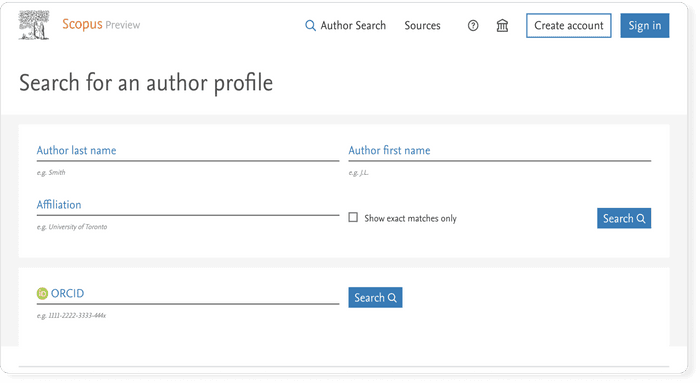
Web of Science also known as Web of Knowledge is the second big bibliographic database. Usually, academic institutions provide either access to Web of Science or Scopus on their campus network for free.
- Coverage: approx. 100 million items
- References: 1.4 billion
- Access options: institutional subscription only
- Provider: Clarivate (formerly Thomson Reuters)
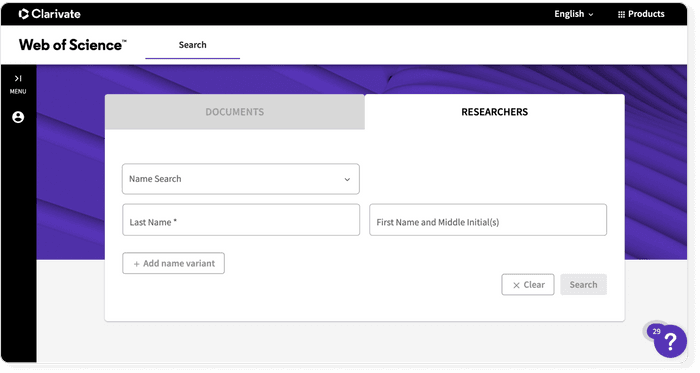
PubMed is the number one resource for anyone looking for literature in medicine or biological sciences. PubMed stores abstracts and bibliographic details of more than 30 million papers and provides full text links to the publisher sites or links to the free PDF on PubMed Central (PMC) .
- Coverage: approx. 35 million items
- Discipline: Medicine and Biological Sciences
- Access options: free
- Provider: NIH
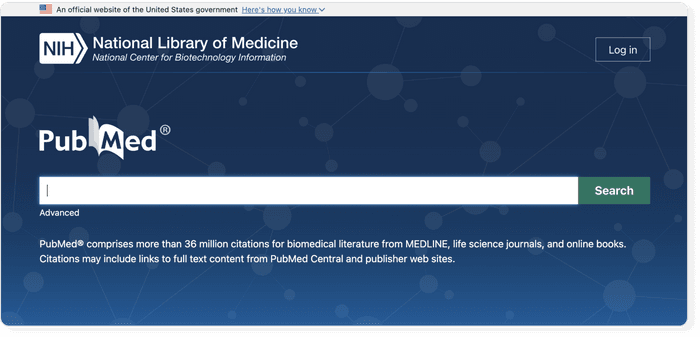
For education sciences, ERIC is the number one destination. ERIC stands for Education Resources Information Center, and is a database that specifically hosts education-related literature.
- Coverage: approx. 1.6 million items
- Discipline: Education
- Provider: U.S. Department of Education
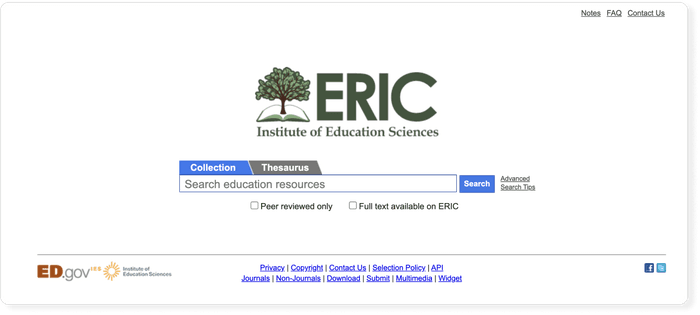
IEEE Xplore is the leading academic database in the field of engineering and computer science. It's not only journal articles, but also conference papers, standards and books that can be search for.
- Coverage: approx. 6 million items
- Discipline: Engineering
- Provider: IEEE (Institute of Electrical and Electronics Engineers)
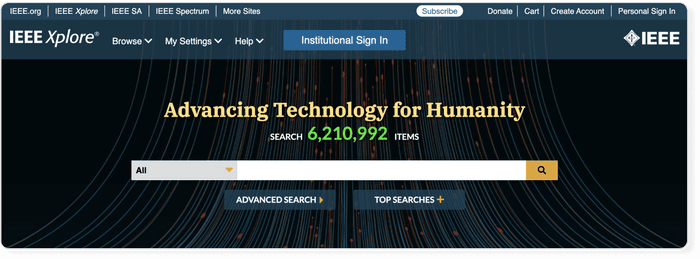
ScienceDirect is the gateway to the millions of academic articles published by Elsevier, 1.4 million of which are open access. Journals and books can be searched via a single interface.
- Coverage: approx. 19.5 million items
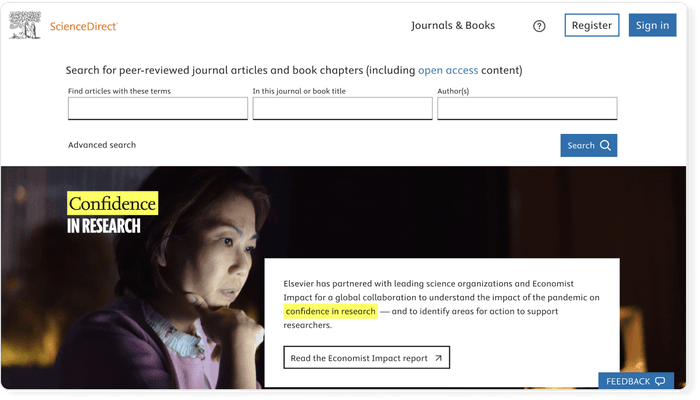
The DOAJ is an open-access academic database that can be accessed and searched for free.
- Coverage: over 8 million records
- Provider: DOAJ
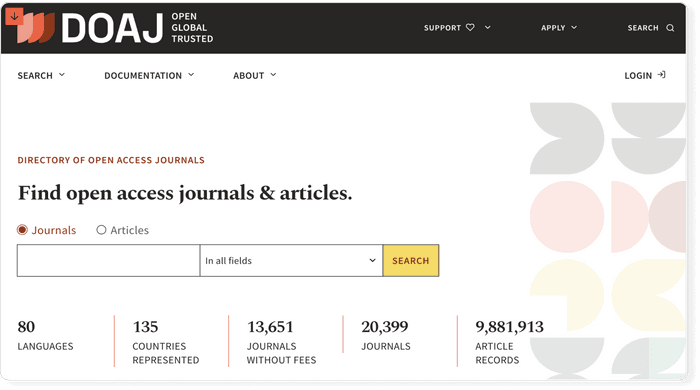
JSTOR is another great resource to find research papers. Any article published before 1924 in the United States is available for free and JSTOR also offers scholarships for independent researchers.
- Coverage: more than 12 million items
- Provider: ITHAKA
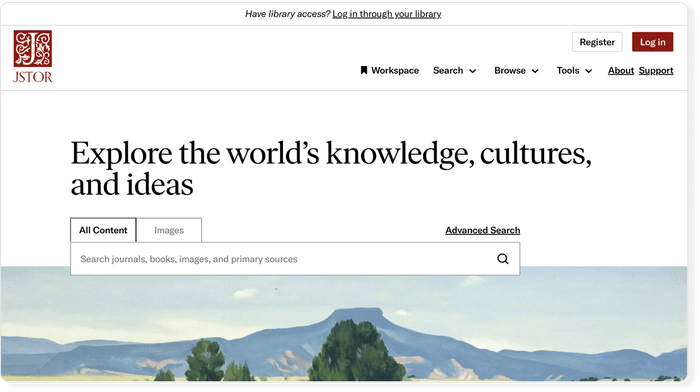
Start using a reference manager like Paperpile to save, organize, and cite your references. Paperpile integrates with PubMed and many popular databases, so you can save references and PDFs directly to your library using the Paperpile buttons:
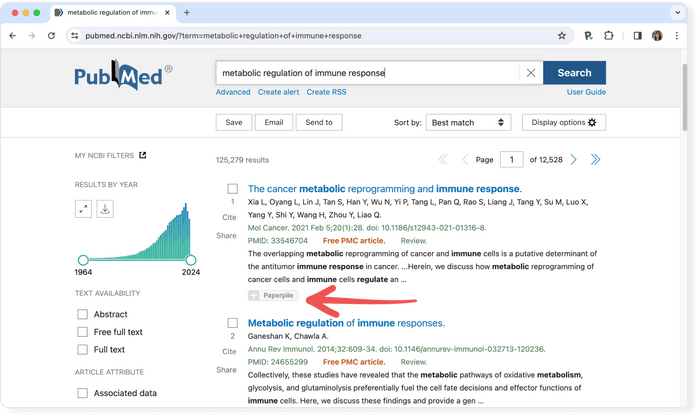
Scopus is one of the two big commercial, bibliographic databases that cover scholarly literature from almost any discipline. Beside searching for research articles, Scopus also provides academic journal rankings, author profiles, and an h-index calculator .
PubMed is the number one resource for anyone looking for literature in medicine or biological sciences. PubMed stores abstracts and bibliographic details of more than 30 million papers and provides full text links to the publisher sites or links to the free PDF on PubMed Central (PMC)
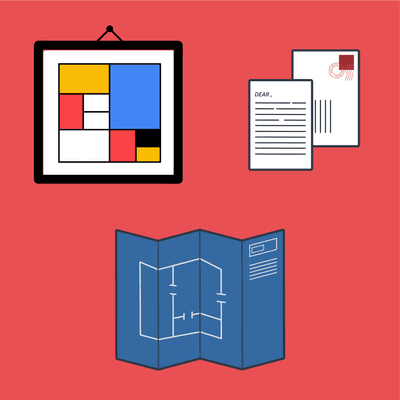
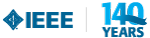
is Mainsite
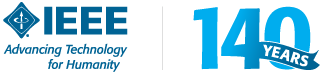
- Search all IEEE websites
- Mission and vision
- IEEE at a glance
- IEEE Strategic Plan
- Organization of IEEE
- Diversity, Equity, & Inclusion
- Organizational Ethics
- Annual Report
- History of IEEE
- Volunteer resources
- IEEE Corporate Awards Program
- Financials and Statistics
- IEEE Future Directions
- IEEE for Industry (Corporations, Government, Individuals)
IEEE Climate Change
- Humanitarian and Philanthropic Opportunities
- Select an option
- Get the latest news
- Access volunteer resources (Code of Ethics, financial forms, tools and templates, and more)
- Find IEEE locations
- Get help from the IEEE Support Center
- Recover your IEEE Account username and password
- Learn about the IEEE Awards program and submit nomination
- View IEEE's organizational structure and leadership
- Apply for jobs at IEEE
- See the history of IEEE
- Learn more about Diversity, Equity & Inclusion at IEEE
- Join an IEEE Society
- Renew your membership
- Member benefits
- IEEE Contact Center
- Connect locally
- Memberships and Subscriptions Catalog
- Member insurance and discounts
- Member Grade Elevation
- Get your company engaged
- Access your Account
- Learn about membership dues
- Learn about Women in Engineering (WIE)
- Access IEEE member email
- Find information on IEEE Fellows
- Access the IEEE member directory
- Learn about the Member-Get-a-Member program
- Learn about IEEE Potentials magazine
- Learn about Student membership
- Affinity groups
- IEEE Societies
- Technical Councils
- Technical Communities
- Geographic Activities
- Working groups
- IEEE Regions
- IEEE Collabratec®
- IEEE Resource Centers
IEEE DataPort
- See the IEEE Regions
- View the MGA Operations Manual
- Find information on IEEE Technical Activities
- Get IEEE Chapter resources
- Find IEEE Sections, Chapters, Student Branches, and other communities
- Learn how to create an IEEE Student Chapter
- Upcoming conferences
- IEEE Meetings, Conferences & Events (MCE)
- IEEE Conference Application
- IEEE Conference Organizer Education Program
- See benefits of authoring a conference paper
- Search for 2025 conferences
- Search for 2024 conferences
- Find conference organizer resources
- Register a conference
- Publish conference papers
- Manage conference finances
- Learn about IEEE Meetings, Conferences & Events (MCE)
- Visit the IEEE SA site
- Become a member of the IEEE SA
- Find information on the IEEE Registration Authority
- Obtain a MAC, OUI, or Ethernet address
- Access the IEEE 802.11™ WLAN standard
- Purchase standards
- Get free select IEEE standards
- Purchase standards subscriptions on IEEE Xplore®
- Get involved with standards development
- Find a working group
- Find information on IEEE 802.11™
- Access the National Electrical Safety Code® (NESC®)
- Find MAC, OUI, and Ethernet addresses from Registration Authority (regauth)
- Get free IEEE standards
- Learn more about the IEEE Standards Association
- View Software and Systems Engineering Standards
- IEEE Xplore® Digital Library
- Subscription options
- IEEE Spectrum
- The Institute
Proceedings of the IEEE
- IEEE Access®
- Author resources
- Get an IEEE Xplore Digital Library trial for IEEE members
- Review impact factors of IEEE journals
- Request access to the IEEE Thesaurus and Taxonomy
- Access the IEEE copyright form
- Find article templates in Word and LaTeX formats
- Get author education resources
- Visit the IEEE Xplore digital library
- Find Author Digital Tools for IEEE paper submission
- Review the IEEE plagiarism policy
- Get information about all stages of publishing with IEEE
- IEEE Learning Network (ILN)
- IEEE Credentialing Program
- Pre-university
- IEEE-Eta Kappa Nu
- Accreditation
- Access continuing education courses on the IEEE Learning Network
- Find STEM education resources on TryEngineering.org
- Learn about the TryEngineering Summer Institute for high school students
- Explore university education program resources
- Access pre-university STEM education resources
- Learn about IEEE certificates and how to offer them
- Find information about the IEEE-Eta Kappa Nu honor society
- Learn about resources for final-year engineering projects
- Access career resources
Publications
Ieee provides a wide range of quality publications that make the exchange of technical knowledge and information possible among technology professionals..
Expand All | Collapse All
- > Get an IEEE Xplore Digital Library trial for IEEE members
- > Review impact factors of IEEE journals
- > Access the IEEE thesaurus and taxonomy
- > Find article templates in Word and LaTeX formats
- > Get author education resources
- > Visit the IEEE Xplore Digital Library
- > Learn more about IEEE author tools
- > Review the IEEE plagiarism policy
- > Get information about all stages of publishing with IEEE

Why choose IEEE publications?
IEEE publishes the leading journals, transactions, letters, and magazines in electrical engineering, computing, biotechnology, telecommunications, power and energy, and dozens of other technologies.
In addition, IEEE publishes more than 1,800 leading-edge conference proceedings every year, which are recognized by academia and industry worldwide as the most vital collection of consolidated published papers in electrical engineering, computer science, and related fields.
Spotlight on IEEE publications
Ieee xplore ®.

- About IEEE Xplore
- Visit the IEEE Xplore Digital Library
- See how to purchase articles and standards
- Find support and training
- Browse popular content
- Sign up for a free trial
IEEE Spectrum Magazine
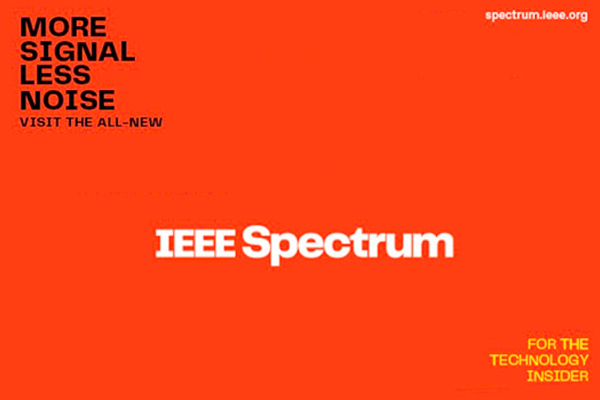
- Visit the IEEE Spectrum website
- Visit the Institute for IEEE member news
IEEE Access

- Visit IEEE Access
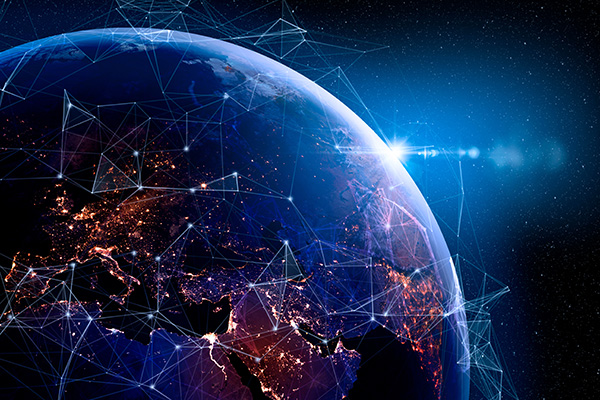
- See recent issues
Benefits of publishing
Authors: why publish with ieee.
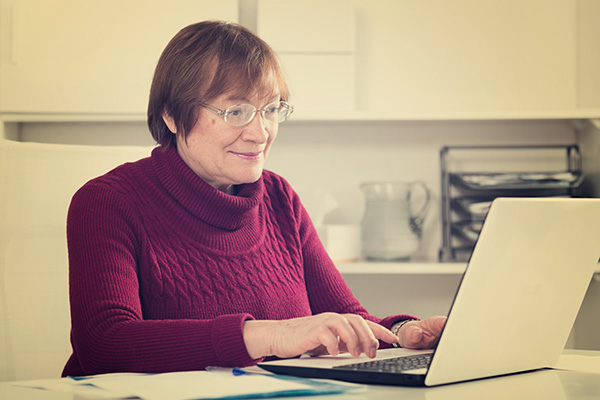
- PSPB Accomplishments in 2023 (PDF, 228 KB)
- IEEE statement of support for Open Science
- IEEE signs San Francisco Declaration on Research Assessment (DORA)
- Read about how IEEE journals maintain top citation rankings
Open Access Solutions
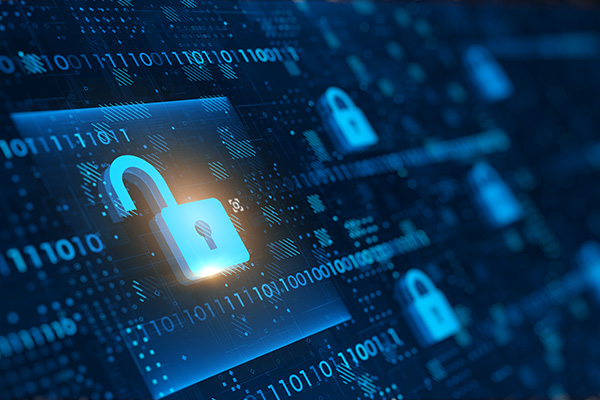
- Visit IEEE Open
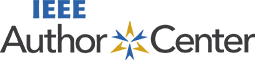
Visit the IEEE Author Center
Find author resources >
- > IEEE Collabratec ®
- > Choosing a journal
- > Writing
- > Author Tools
- > How to Publish with IEEE (English) (PPT, 3 MB)
- > How to Publish with IEEE (Chinese) (PPT, 3 MB)
- > Benefits of Publishing with IEEE (PPT, 7 MB)
- > View author tutorial videos
- Read the IEEE statement on appropriate use of bibliometric indicators
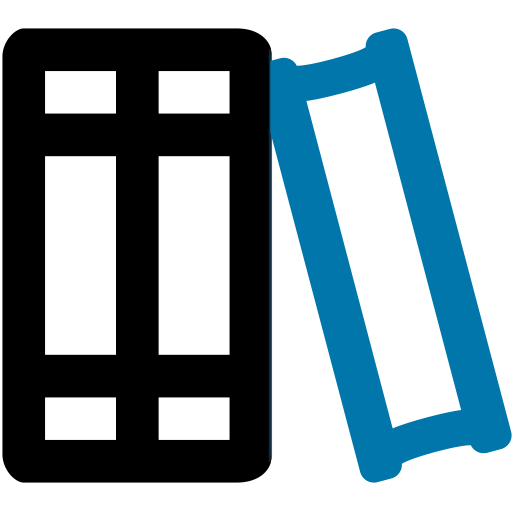
Publication types and subscription options
- Journal and magazine subscriptions
- Digital library subscriptions
- Buy individual articles from IEEE Xplore
For organizations:
- Browse IEEE subscriptions
- Get institutional access
- Subscribe through your local IEEE account manager
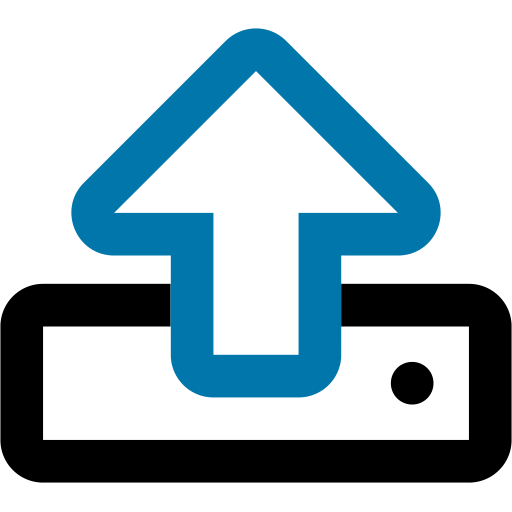
Publishing information
IEEE publishing makes the exchange of technical knowledge possible with the highest quality and the greatest impact.
- Open access publishing options
- Intellectual Property Rights (IPR)
- Reprints of articles
- Services for IEEE organizations
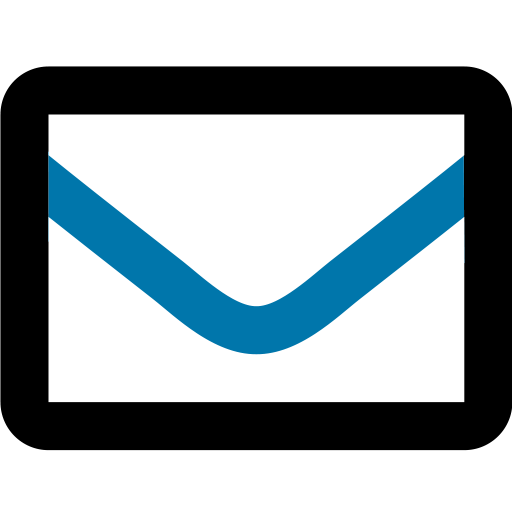
Contact information
- Contact IEEE Publications
- About the Publication Services & Products Board
Related Information >
Network. collaborate. create with ieee collabratec®..
All within one central hub—with exclusive features for IEEE members.
- Experience IEEE Collabratec
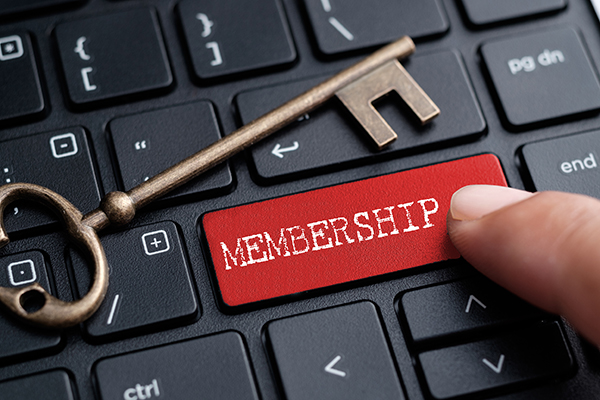
Join/Renew IEEE or a Society
Receive member access to select content, product discounts, and more.
- Review all member benefits
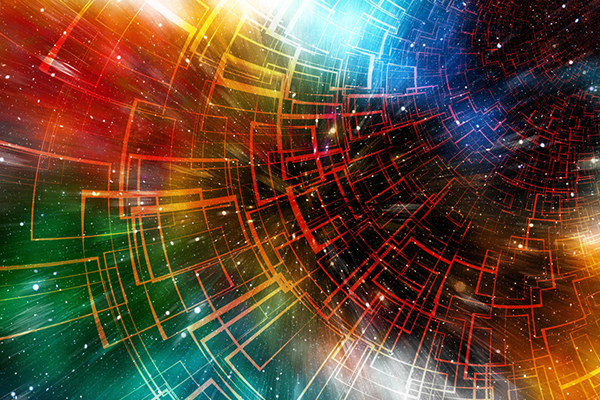
Try this easy-to-use, globally accessible data repository that provides significant benefits to researchers, data analysts, and the global technical community.
- Start learning today
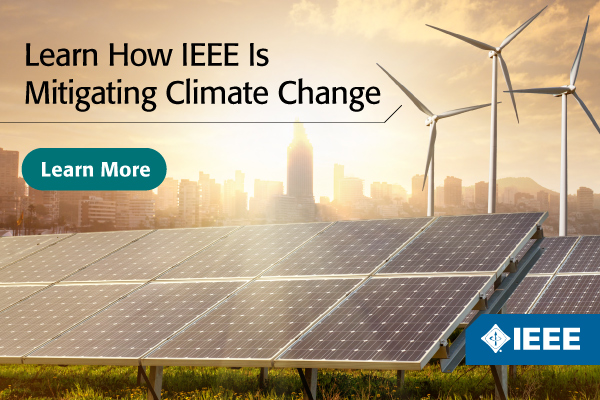
IEEE is committed to helping combat and mitigate the effects of climate change.
- See what's new on the IEEE Climate Change site
- Corrections
Search Help
Get the most out of Google Scholar with some helpful tips on searches, email alerts, citation export, and more.
Finding recent papers
Your search results are normally sorted by relevance, not by date. To find newer articles, try the following options in the left sidebar:
- click "Since Year" to show only recently published papers, sorted by relevance;
- click "Sort by date" to show just the new additions, sorted by date;
- click the envelope icon to have new results periodically delivered by email.
Locating the full text of an article
Abstracts are freely available for most of the articles. Alas, reading the entire article may require a subscription. Here're a few things to try:
- click a library link, e.g., "FindIt@Harvard", to the right of the search result;
- click a link labeled [PDF] to the right of the search result;
- click "All versions" under the search result and check out the alternative sources;
- click "Related articles" or "Cited by" under the search result to explore similar articles.
If you're affiliated with a university, but don't see links such as "FindIt@Harvard", please check with your local library about the best way to access their online subscriptions. You may need to do search from a computer on campus, or to configure your browser to use a library proxy.
Getting better answers
If you're new to the subject, it may be helpful to pick up the terminology from secondary sources. E.g., a Wikipedia article for "overweight" might suggest a Scholar search for "pediatric hyperalimentation".
If the search results are too specific for your needs, check out what they're citing in their "References" sections. Referenced works are often more general in nature.
Similarly, if the search results are too basic for you, click "Cited by" to see newer papers that referenced them. These newer papers will often be more specific.
Explore! There's rarely a single answer to a research question. Click "Related articles" or "Cited by" to see closely related work, or search for author's name and see what else they have written.
Searching Google Scholar
Use the "author:" operator, e.g., author:"d knuth" or author:"donald e knuth".
Put the paper's title in quotations: "A History of the China Sea".
You'll often get better results if you search only recent articles, but still sort them by relevance, not by date. E.g., click "Since 2018" in the left sidebar of the search results page.
To see the absolutely newest articles first, click "Sort by date" in the sidebar. If you use this feature a lot, you may also find it useful to setup email alerts to have new results automatically sent to you.
Note: On smaller screens that don't show the sidebar, these options are available in the dropdown menu labelled "Year" right below the search button.
Select the "Case law" option on the homepage or in the side drawer on the search results page.
It finds documents similar to the given search result.
It's in the side drawer. The advanced search window lets you search in the author, title, and publication fields, as well as limit your search results by date.
Select the "Case law" option and do a keyword search over all jurisdictions. Then, click the "Select courts" link in the left sidebar on the search results page.
Tip: To quickly search a frequently used selection of courts, bookmark a search results page with the desired selection.
Access to articles
For each Scholar search result, we try to find a version of the article that you can read. These access links are labelled [PDF] or [HTML] and appear to the right of the search result. For example:
A paper that you need to read
Access links cover a wide variety of ways in which articles may be available to you - articles that your library subscribes to, open access articles, free-to-read articles from publishers, preprints, articles in repositories, etc.
When you are on a campus network, access links automatically include your library subscriptions and direct you to subscribed versions of articles. On-campus access links cover subscriptions from primary publishers as well as aggregators.
Off-campus access
Off-campus access links let you take your library subscriptions with you when you are at home or traveling. You can read subscribed articles when you are off-campus just as easily as when you are on-campus. Off-campus access links work by recording your subscriptions when you visit Scholar while on-campus, and looking up the recorded subscriptions later when you are off-campus.
We use the recorded subscriptions to provide you with the same subscribed access links as you see on campus. We also indicate your subscription access to participating publishers so that they can allow you to read the full-text of these articles without logging in or using a proxy. The recorded subscription information expires after 30 days and is automatically deleted.
In addition to Google Scholar search results, off-campus access links can also appear on articles from publishers participating in the off-campus subscription access program. Look for links labeled [PDF] or [HTML] on the right hand side of article pages.
Anne Author , John Doe , Jane Smith , Someone Else
In this fascinating paper, we investigate various topics that would be of interest to you. We also describe new methods relevant to your project, and attempt to address several questions which you would also like to know the answer to. Lastly, we analyze …
You can disable off-campus access links on the Scholar settings page . Disabling off-campus access links will turn off recording of your library subscriptions. It will also turn off indicating subscription access to participating publishers. Once off-campus access links are disabled, you may need to identify and configure an alternate mechanism (e.g., an institutional proxy or VPN) to access your library subscriptions while off-campus.
Email Alerts
Do a search for the topic of interest, e.g., "M Theory"; click the envelope icon in the sidebar of the search results page; enter your email address, and click "Create alert". We'll then periodically email you newly published papers that match your search criteria.
No, you can enter any email address of your choice. If the email address isn't a Google account or doesn't match your Google account, then we'll email you a verification link, which you'll need to click to start receiving alerts.
This works best if you create a public profile , which is free and quick to do. Once you get to the homepage with your photo, click "Follow" next to your name, select "New citations to my articles", and click "Done". We will then email you when we find new articles that cite yours.
Search for the title of your paper, e.g., "Anti de Sitter space and holography"; click on the "Cited by" link at the bottom of the search result; and then click on the envelope icon in the left sidebar of the search results page.
First, do a search for your colleague's name, and see if they have a Scholar profile. If they do, click on it, click the "Follow" button next to their name, select "New articles by this author", and click "Done".
If they don't have a profile, do a search by author, e.g., [author:s-hawking], and click on the mighty envelope in the left sidebar of the search results page. If you find that several different people share the same name, you may need to add co-author names or topical keywords to limit results to the author you wish to follow.
We send the alerts right after we add new papers to Google Scholar. This usually happens several times a week, except that our search robots meticulously observe holidays.
There's a link to cancel the alert at the bottom of every notification email.
If you created alerts using a Google account, you can manage them all here . If you're not using a Google account, you'll need to unsubscribe from the individual alerts and subscribe to the new ones.
Google Scholar library
Google Scholar library is your personal collection of articles. You can save articles right off the search page, organize them by adding labels, and use the power of Scholar search to quickly find just the one you want - at any time and from anywhere. You decide what goes into your library, and we’ll keep the links up to date.
You get all the goodies that come with Scholar search results - links to PDF and to your university's subscriptions, formatted citations, citing articles, and more!
Library help
Find the article you want to add in Google Scholar and click the “Save” button under the search result.
Click “My library” at the top of the page or in the side drawer to view all articles in your library. To search the full text of these articles, enter your query as usual in the search box.
Find the article you want to remove, and then click the “Delete” button under it.
- To add a label to an article, find the article in your library, click the “Label” button under it, select the label you want to apply, and click “Done”.
- To view all the articles with a specific label, click the label name in the left sidebar of your library page.
- To remove a label from an article, click the “Label” button under it, deselect the label you want to remove, and click “Done”.
- To add, edit, or delete labels, click “Manage labels” in the left column of your library page.
Only you can see the articles in your library. If you create a Scholar profile and make it public, then the articles in your public profile (and only those articles) will be visible to everyone.
Your profile contains all the articles you have written yourself. It’s a way to present your work to others, as well as to keep track of citations to it. Your library is a way to organize the articles that you’d like to read or cite, not necessarily the ones you’ve written.
Citation Export
Click the "Cite" button under the search result and then select your bibliography manager at the bottom of the popup. We currently support BibTeX, EndNote, RefMan, and RefWorks.
Err, no, please respect our robots.txt when you access Google Scholar using automated software. As the wearers of crawler's shoes and webmaster's hat, we cannot recommend adherence to web standards highly enough.
Sorry, we're unable to provide bulk access. You'll need to make an arrangement directly with the source of the data you're interested in. Keep in mind that a lot of the records in Google Scholar come from commercial subscription services.
Sorry, we can only show up to 1,000 results for any particular search query. Try a different query to get more results.
Content Coverage
Google Scholar includes journal and conference papers, theses and dissertations, academic books, pre-prints, abstracts, technical reports and other scholarly literature from all broad areas of research. You'll find works from a wide variety of academic publishers, professional societies and university repositories, as well as scholarly articles available anywhere across the web. Google Scholar also includes court opinions and patents.
We index research articles and abstracts from most major academic publishers and repositories worldwide, including both free and subscription sources. To check current coverage of a specific source in Google Scholar, search for a sample of their article titles in quotes.
While we try to be comprehensive, it isn't possible to guarantee uninterrupted coverage of any particular source. We index articles from sources all over the web and link to these websites in our search results. If one of these websites becomes unavailable to our search robots or to a large number of web users, we have to remove it from Google Scholar until it becomes available again.
Our meticulous search robots generally try to index every paper from every website they visit, including most major sources and also many lesser known ones.
That said, Google Scholar is primarily a search of academic papers. Shorter articles, such as book reviews, news sections, editorials, announcements and letters, may or may not be included. Untitled documents and documents without authors are usually not included. Website URLs that aren't available to our search robots or to the majority of web users are, obviously, not included either. Nor do we include websites that require you to sign up for an account, install a browser plugin, watch four colorful ads, and turn around three times and say coo-coo before you can read the listing of titles scanned at 10 DPI... You get the idea, we cover academic papers from sensible websites.
That's usually because we index many of these papers from other websites, such as the websites of their primary publishers. The "site:" operator currently only searches the primary version of each paper.
It could also be that the papers are located on examplejournals.gov, not on example.gov. Please make sure you're searching for the "right" website.
That said, the best way to check coverage of a specific source is to search for a sample of their papers using the title of the paper.
Ahem, we index papers, not journals. You should also ask about our coverage of universities, research groups, proteins, seminal breakthroughs, and other dimensions that are of interest to users. All such questions are best answered by searching for a statistical sample of papers that has the property of interest - journal, author, protein, etc. Many coverage comparisons are available if you search for [allintitle:"google scholar"], but some of them are more statistically valid than others.
Currently, Google Scholar allows you to search and read published opinions of US state appellate and supreme court cases since 1950, US federal district, appellate, tax and bankruptcy courts since 1923 and US Supreme Court cases since 1791. In addition, it includes citations for cases cited by indexed opinions or journal articles which allows you to find influential cases (usually older or international) which are not yet online or publicly available.
Legal opinions in Google Scholar are provided for informational purposes only and should not be relied on as a substitute for legal advice from a licensed lawyer. Google does not warrant that the information is complete or accurate.
We normally add new papers several times a week. However, updates to existing records take 6-9 months to a year or longer, because in order to update our records, we need to first recrawl them from the source website. For many larger websites, the speed at which we can update their records is limited by the crawl rate that they allow.
Inclusion and Corrections
We apologize, and we assure you the error was unintentional. Automated extraction of information from articles in diverse fields can be tricky, so an error sometimes sneaks through.
Please write to the owner of the website where the erroneous search result is coming from, and encourage them to provide correct bibliographic data to us, as described in the technical guidelines . Once the data is corrected on their website, it usually takes 6-9 months to a year or longer for it to be updated in Google Scholar. We appreciate your help and your patience.
If you can't find your papers when you search for them by title and by author, please refer your publisher to our technical guidelines .
You can also deposit your papers into your institutional repository or put their PDF versions on your personal website, but please follow your publisher's requirements when you do so. See our technical guidelines for more details on the inclusion process.
We normally add new papers several times a week; however, it might take us some time to crawl larger websites, and corrections to already included papers can take 6-9 months to a year or longer.
Google Scholar generally reflects the state of the web as it is currently visible to our search robots and to the majority of users. When you're searching for relevant papers to read, you wouldn't want it any other way!
If your citation counts have gone down, chances are that either your paper or papers that cite it have either disappeared from the web entirely, or have become unavailable to our search robots, or, perhaps, have been reformatted in a way that made it difficult for our automated software to identify their bibliographic data and references. If you wish to correct this, you'll need to identify the specific documents with indexing problems and ask your publisher to fix them. Please refer to the technical guidelines .
Please do let us know . Please include the URL for the opinion, the corrected information and a source where we can verify the correction.
We're only able to make corrections to court opinions that are hosted on our own website. For corrections to academic papers, books, dissertations and other third-party material, click on the search result in question and contact the owner of the website where the document came from. For corrections to books from Google Book Search, click on the book's title and locate the link to provide feedback at the bottom of the book's page.
General Questions
These are articles which other scholarly articles have referred to, but which we haven't found online. To exclude them from your search results, uncheck the "include citations" box on the left sidebar.
First, click on links labeled [PDF] or [HTML] to the right of the search result's title. Also, check out the "All versions" link at the bottom of the search result.
Second, if you're affiliated with a university, using a computer on campus will often let you access your library's online subscriptions. Look for links labeled with your library's name to the right of the search result's title. Also, see if there's a link to the full text on the publisher's page with the abstract.
Keep in mind that final published versions are often only available to subscribers, and that some articles are not available online at all. Good luck!
Technically, your web browser remembers your settings in a "cookie" on your computer's disk, and sends this cookie to our website along with every search. Check that your browser isn't configured to discard our cookies. Also, check if disabling various proxies or overly helpful privacy settings does the trick. Either way, your settings are stored on your computer, not on our servers, so a long hard look at your browser's preferences or internet options should help cure the machine's forgetfulness.
Not even close. That phrase is our acknowledgement that much of scholarly research involves building on what others have already discovered. It's taken from Sir Isaac Newton's famous quote, "If I have seen further, it is by standing on the shoulders of giants."
- Privacy & Terms
How to Write and Publish a Research Paper for a Peer-Reviewed Journal
- Open access
- Published: 30 April 2020
- Volume 36 , pages 909–913, ( 2021 )
Cite this article
You have full access to this open access article
- Clara Busse ORCID: orcid.org/0000-0002-0178-1000 1 &
- Ella August ORCID: orcid.org/0000-0001-5151-1036 1 , 2
279k Accesses
15 Citations
709 Altmetric
Explore all metrics
Communicating research findings is an essential step in the research process. Often, peer-reviewed journals are the forum for such communication, yet many researchers are never taught how to write a publishable scientific paper. In this article, we explain the basic structure of a scientific paper and describe the information that should be included in each section. We also identify common pitfalls for each section and recommend strategies to avoid them. Further, we give advice about target journal selection and authorship. In the online resource 1 , we provide an example of a high-quality scientific paper, with annotations identifying the elements we describe in this article.
Similar content being viewed by others
How to Choose the Right Journal
The Point Is…to Publish?
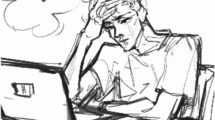
Writing and publishing a scientific paper
Avoid common mistakes on your manuscript.
Introduction
Writing a scientific paper is an important component of the research process, yet researchers often receive little formal training in scientific writing. This is especially true in low-resource settings. In this article, we explain why choosing a target journal is important, give advice about authorship, provide a basic structure for writing each section of a scientific paper, and describe common pitfalls and recommendations for each section. In the online resource 1 , we also include an annotated journal article that identifies the key elements and writing approaches that we detail here. Before you begin your research, make sure you have ethical clearance from all relevant ethical review boards.
Select a Target Journal Early in the Writing Process
We recommend that you select a “target journal” early in the writing process; a “target journal” is the journal to which you plan to submit your paper. Each journal has a set of core readers and you should tailor your writing to this readership. For example, if you plan to submit a manuscript about vaping during pregnancy to a pregnancy-focused journal, you will need to explain what vaping is because readers of this journal may not have a background in this topic. However, if you were to submit that same article to a tobacco journal, you would not need to provide as much background information about vaping.
Information about a journal’s core readership can be found on its website, usually in a section called “About this journal” or something similar. For example, the Journal of Cancer Education presents such information on the “Aims and Scope” page of its website, which can be found here: https://www.springer.com/journal/13187/aims-and-scope .
Peer reviewer guidelines from your target journal are an additional resource that can help you tailor your writing to the journal and provide additional advice about crafting an effective article [ 1 ]. These are not always available, but it is worth a quick web search to find out.
Identify Author Roles Early in the Process
Early in the writing process, identify authors, determine the order of authors, and discuss the responsibilities of each author. Standard author responsibilities have been identified by The International Committee of Medical Journal Editors (ICMJE) [ 2 ]. To set clear expectations about each team member’s responsibilities and prevent errors in communication, we also suggest outlining more detailed roles, such as who will draft each section of the manuscript, write the abstract, submit the paper electronically, serve as corresponding author, and write the cover letter. It is best to formalize this agreement in writing after discussing it, circulating the document to the author team for approval. We suggest creating a title page on which all authors are listed in the agreed-upon order. It may be necessary to adjust authorship roles and order during the development of the paper. If a new author order is agreed upon, be sure to update the title page in the manuscript draft.
In the case where multiple papers will result from a single study, authors should discuss who will author each paper. Additionally, authors should agree on a deadline for each paper and the lead author should take responsibility for producing an initial draft by this deadline.
Structure of the Introduction Section
The introduction section should be approximately three to five paragraphs in length. Look at examples from your target journal to decide the appropriate length. This section should include the elements shown in Fig. 1 . Begin with a general context, narrowing to the specific focus of the paper. Include five main elements: why your research is important, what is already known about the topic, the “gap” or what is not yet known about the topic, why it is important to learn the new information that your research adds, and the specific research aim(s) that your paper addresses. Your research aim should address the gap you identified. Be sure to add enough background information to enable readers to understand your study. Table 1 provides common introduction section pitfalls and recommendations for addressing them.
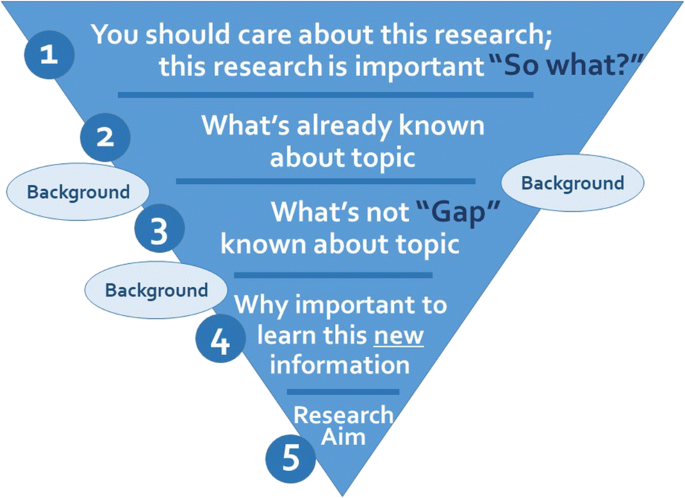
The main elements of the introduction section of an original research article. Often, the elements overlap
Methods Section
The purpose of the methods section is twofold: to explain how the study was done in enough detail to enable its replication and to provide enough contextual detail to enable readers to understand and interpret the results. In general, the essential elements of a methods section are the following: a description of the setting and participants, the study design and timing, the recruitment and sampling, the data collection process, the dataset, the dependent and independent variables, the covariates, the analytic approach for each research objective, and the ethical approval. The hallmark of an exemplary methods section is the justification of why each method was used. Table 2 provides common methods section pitfalls and recommendations for addressing them.
Results Section
The focus of the results section should be associations, or lack thereof, rather than statistical tests. Two considerations should guide your writing here. First, the results should present answers to each part of the research aim. Second, return to the methods section to ensure that the analysis and variables for each result have been explained.
Begin the results section by describing the number of participants in the final sample and details such as the number who were approached to participate, the proportion who were eligible and who enrolled, and the number of participants who dropped out. The next part of the results should describe the participant characteristics. After that, you may organize your results by the aim or by putting the most exciting results first. Do not forget to report your non-significant associations. These are still findings.
Tables and figures capture the reader’s attention and efficiently communicate your main findings [ 3 ]. Each table and figure should have a clear message and should complement, rather than repeat, the text. Tables and figures should communicate all salient details necessary for a reader to understand the findings without consulting the text. Include information on comparisons and tests, as well as information about the sample and timing of the study in the title, legend, or in a footnote. Note that figures are often more visually interesting than tables, so if it is feasible to make a figure, make a figure. To avoid confusing the reader, either avoid abbreviations in tables and figures, or define them in a footnote. Note that there should not be citations in the results section and you should not interpret results here. Table 3 provides common results section pitfalls and recommendations for addressing them.
Discussion Section
Opposite the introduction section, the discussion should take the form of a right-side-up triangle beginning with interpretation of your results and moving to general implications (Fig. 2 ). This section typically begins with a restatement of the main findings, which can usually be accomplished with a few carefully-crafted sentences.
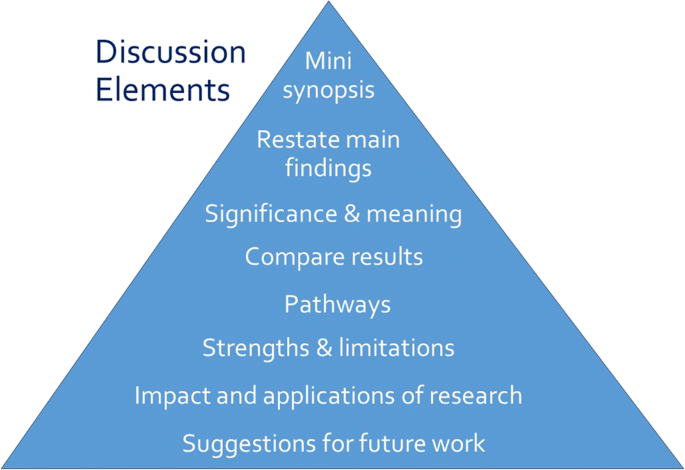
Major elements of the discussion section of an original research article. Often, the elements overlap
Next, interpret the meaning or explain the significance of your results, lifting the reader’s gaze from the study’s specific findings to more general applications. Then, compare these study findings with other research. Are these findings in agreement or disagreement with those from other studies? Does this study impart additional nuance to well-accepted theories? Situate your findings within the broader context of scientific literature, then explain the pathways or mechanisms that might give rise to, or explain, the results.
Journals vary in their approach to strengths and limitations sections: some are embedded paragraphs within the discussion section, while some mandate separate section headings. Keep in mind that every study has strengths and limitations. Candidly reporting yours helps readers to correctly interpret your research findings.
The next element of the discussion is a summary of the potential impacts and applications of the research. Should these results be used to optimally design an intervention? Does the work have implications for clinical protocols or public policy? These considerations will help the reader to further grasp the possible impacts of the presented work.
Finally, the discussion should conclude with specific suggestions for future work. Here, you have an opportunity to illuminate specific gaps in the literature that compel further study. Avoid the phrase “future research is necessary” because the recommendation is too general to be helpful to readers. Instead, provide substantive and specific recommendations for future studies. Table 4 provides common discussion section pitfalls and recommendations for addressing them.
Follow the Journal’s Author Guidelines
After you select a target journal, identify the journal’s author guidelines to guide the formatting of your manuscript and references. Author guidelines will often (but not always) include instructions for titles, cover letters, and other components of a manuscript submission. Read the guidelines carefully. If you do not follow the guidelines, your article will be sent back to you.
Finally, do not submit your paper to more than one journal at a time. Even if this is not explicitly stated in the author guidelines of your target journal, it is considered inappropriate and unprofessional.
Your title should invite readers to continue reading beyond the first page [ 4 , 5 ]. It should be informative and interesting. Consider describing the independent and dependent variables, the population and setting, the study design, the timing, and even the main result in your title. Because the focus of the paper can change as you write and revise, we recommend you wait until you have finished writing your paper before composing the title.
Be sure that the title is useful for potential readers searching for your topic. The keywords you select should complement those in your title to maximize the likelihood that a researcher will find your paper through a database search. Avoid using abbreviations in your title unless they are very well known, such as SNP, because it is more likely that someone will use a complete word rather than an abbreviation as a search term to help readers find your paper.
After you have written a complete draft, use the checklist (Fig. 3 ) below to guide your revisions and editing. Additional resources are available on writing the abstract and citing references [ 5 ]. When you feel that your work is ready, ask a trusted colleague or two to read the work and provide informal feedback. The box below provides a checklist that summarizes the key points offered in this article.
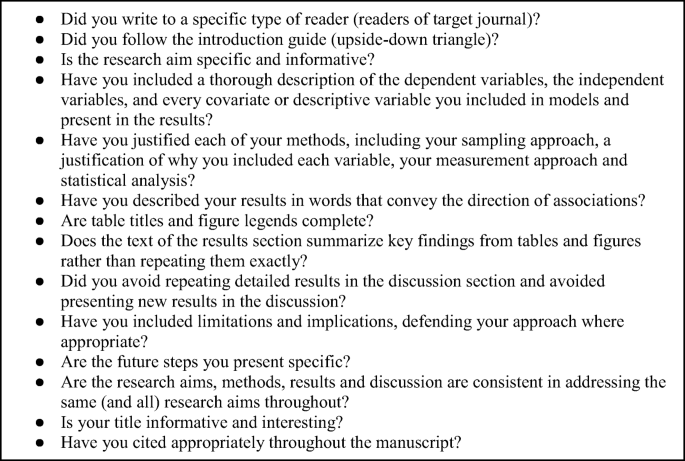
Checklist for manuscript quality
Data Availability
Michalek AM (2014) Down the rabbit hole…advice to reviewers. J Cancer Educ 29:4–5
Article Google Scholar
International Committee of Medical Journal Editors. Defining the role of authors and contributors: who is an author? http://www.icmje.org/recommendations/browse/roles-and-responsibilities/defining-the-role-of-authosrs-and-contributors.html . Accessed 15 January, 2020
Vetto JT (2014) Short and sweet: a short course on concise medical writing. J Cancer Educ 29(1):194–195
Brett M, Kording K (2017) Ten simple rules for structuring papers. PLoS ComputBiol. https://doi.org/10.1371/journal.pcbi.1005619
Lang TA (2017) Writing a better research article. J Public Health Emerg. https://doi.org/10.21037/jphe.2017.11.06
Download references
Acknowledgments
Ella August is grateful to the Sustainable Sciences Institute for mentoring her in training researchers on writing and publishing their research.
Code Availability
Not applicable.
Author information
Authors and affiliations.
Department of Maternal and Child Health, University of North Carolina Gillings School of Global Public Health, 135 Dauer Dr, 27599, Chapel Hill, NC, USA
Clara Busse & Ella August
Department of Epidemiology, University of Michigan School of Public Health, 1415 Washington Heights, Ann Arbor, MI, 48109-2029, USA
Ella August
You can also search for this author in PubMed Google Scholar
Corresponding author
Correspondence to Ella August .
Ethics declarations
Conflicts of interests.
The authors declare that they have no conflict of interest.
Additional information
Publisher’s note.
Springer Nature remains neutral with regard to jurisdictional claims in published maps and institutional affiliations.
Electronic supplementary material
(PDF 362 kb)
Rights and permissions
Open Access This article is licensed under a Creative Commons Attribution 4.0 International License, which permits use, sharing, adaptation, distribution and reproduction in any medium or format, as long as you give appropriate credit to the original author(s) and the source, provide a link to the Creative Commons licence, and indicate if changes were made. The images or other third party material in this article are included in the article's Creative Commons licence, unless indicated otherwise in a credit line to the material. If material is not included in the article's Creative Commons licence and your intended use is not permitted by statutory regulation or exceeds the permitted use, you will need to obtain permission directly from the copyright holder. To view a copy of this licence, visit http://creativecommons.org/licenses/by/4.0/ .
Reprints and permissions
About this article
Busse, C., August, E. How to Write and Publish a Research Paper for a Peer-Reviewed Journal. J Canc Educ 36 , 909–913 (2021). https://doi.org/10.1007/s13187-020-01751-z
Download citation
Published : 30 April 2020
Issue Date : October 2021
DOI : https://doi.org/10.1007/s13187-020-01751-z
Share this article
Anyone you share the following link with will be able to read this content:
Sorry, a shareable link is not currently available for this article.
Provided by the Springer Nature SharedIt content-sharing initiative
- Manuscripts
- Scientific writing
- Find a journal
- Publish with us
- Track your research
“The only truly modern academic research engine”
Oa.mg is a search engine for academic papers, specialising in open access. we have over 250 million papers in our index..

An official website of the United States government
The .gov means it’s official. Federal government websites often end in .gov or .mil. Before sharing sensitive information, make sure you’re on a federal government site.
The site is secure. The https:// ensures that you are connecting to the official website and that any information you provide is encrypted and transmitted securely.
- Publications
- Account settings
Preview improvements coming to the PMC website in October 2024. Learn More or Try it out now .
- Advanced Search
- Journal List

How to Write and Publish a Research Paper for a Peer-Reviewed Journal
Clara busse.
1 Department of Maternal and Child Health, University of North Carolina Gillings School of Global Public Health, 135 Dauer Dr, 27599 Chapel Hill, NC USA
Ella August
2 Department of Epidemiology, University of Michigan School of Public Health, 1415 Washington Heights, Ann Arbor, MI 48109-2029 USA
Associated Data
Communicating research findings is an essential step in the research process. Often, peer-reviewed journals are the forum for such communication, yet many researchers are never taught how to write a publishable scientific paper. In this article, we explain the basic structure of a scientific paper and describe the information that should be included in each section. We also identify common pitfalls for each section and recommend strategies to avoid them. Further, we give advice about target journal selection and authorship. In the online resource 1 , we provide an example of a high-quality scientific paper, with annotations identifying the elements we describe in this article.
Electronic supplementary material
The online version of this article (10.1007/s13187-020-01751-z) contains supplementary material, which is available to authorized users.
Introduction
Writing a scientific paper is an important component of the research process, yet researchers often receive little formal training in scientific writing. This is especially true in low-resource settings. In this article, we explain why choosing a target journal is important, give advice about authorship, provide a basic structure for writing each section of a scientific paper, and describe common pitfalls and recommendations for each section. In the online resource 1 , we also include an annotated journal article that identifies the key elements and writing approaches that we detail here. Before you begin your research, make sure you have ethical clearance from all relevant ethical review boards.
Select a Target Journal Early in the Writing Process
We recommend that you select a “target journal” early in the writing process; a “target journal” is the journal to which you plan to submit your paper. Each journal has a set of core readers and you should tailor your writing to this readership. For example, if you plan to submit a manuscript about vaping during pregnancy to a pregnancy-focused journal, you will need to explain what vaping is because readers of this journal may not have a background in this topic. However, if you were to submit that same article to a tobacco journal, you would not need to provide as much background information about vaping.
Information about a journal’s core readership can be found on its website, usually in a section called “About this journal” or something similar. For example, the Journal of Cancer Education presents such information on the “Aims and Scope” page of its website, which can be found here: https://www.springer.com/journal/13187/aims-and-scope .
Peer reviewer guidelines from your target journal are an additional resource that can help you tailor your writing to the journal and provide additional advice about crafting an effective article [ 1 ]. These are not always available, but it is worth a quick web search to find out.
Identify Author Roles Early in the Process
Early in the writing process, identify authors, determine the order of authors, and discuss the responsibilities of each author. Standard author responsibilities have been identified by The International Committee of Medical Journal Editors (ICMJE) [ 2 ]. To set clear expectations about each team member’s responsibilities and prevent errors in communication, we also suggest outlining more detailed roles, such as who will draft each section of the manuscript, write the abstract, submit the paper electronically, serve as corresponding author, and write the cover letter. It is best to formalize this agreement in writing after discussing it, circulating the document to the author team for approval. We suggest creating a title page on which all authors are listed in the agreed-upon order. It may be necessary to adjust authorship roles and order during the development of the paper. If a new author order is agreed upon, be sure to update the title page in the manuscript draft.
In the case where multiple papers will result from a single study, authors should discuss who will author each paper. Additionally, authors should agree on a deadline for each paper and the lead author should take responsibility for producing an initial draft by this deadline.
Structure of the Introduction Section
The introduction section should be approximately three to five paragraphs in length. Look at examples from your target journal to decide the appropriate length. This section should include the elements shown in Fig. 1 . Begin with a general context, narrowing to the specific focus of the paper. Include five main elements: why your research is important, what is already known about the topic, the “gap” or what is not yet known about the topic, why it is important to learn the new information that your research adds, and the specific research aim(s) that your paper addresses. Your research aim should address the gap you identified. Be sure to add enough background information to enable readers to understand your study. Table Table1 1 provides common introduction section pitfalls and recommendations for addressing them.

The main elements of the introduction section of an original research article. Often, the elements overlap
Common introduction section pitfalls and recommendations
Pitfall | Recommendation |
---|---|
Introduction is too generic, not written to specific readers of a designated journal. | Visit your target journal’s website and investigate the journal’s readership. If you are writing for a journal with a more general readership, like PLOS ONE, you should include more background information. A narrower journal, like the Journal of the American Mosquito Control Association, may require less background information because most of its readers have expertise in the subject matter. |
Citations are inadequate to support claims. | If a claim could be debated, it should be supported by one or more citations. To find articles relevant to your research, consider using open-access journals, which are available for anyone to read for free. A list of open-access journals can be found here: . You can also find open-access articles using PubMed Central: |
The research aim is vague. | Be sure that your research aim contains essential details like the setting, population/sample, study design, timing, dependent variable, and independent variables. Using such details, the reader should be able to imagine the analysis you have conducted. |
Methods Section
The purpose of the methods section is twofold: to explain how the study was done in enough detail to enable its replication and to provide enough contextual detail to enable readers to understand and interpret the results. In general, the essential elements of a methods section are the following: a description of the setting and participants, the study design and timing, the recruitment and sampling, the data collection process, the dataset, the dependent and independent variables, the covariates, the analytic approach for each research objective, and the ethical approval. The hallmark of an exemplary methods section is the justification of why each method was used. Table Table2 2 provides common methods section pitfalls and recommendations for addressing them.
Common methods section pitfalls and recommendations
Pitfall | Recommendation |
---|---|
The author only describes methods for one study aim, or part of an aim. |
Be sure to check that the methods describe all aspects of the study reported in the manuscript. |
There is not enough (or any) justification for the methods used. | You must justify your choice of methods because it greatly impacts the interpretation of results. State the methods you used and then defend those decisions. For example, justify why you chose to include the measurements, covariates, and statistical approaches. |
Results Section
The focus of the results section should be associations, or lack thereof, rather than statistical tests. Two considerations should guide your writing here. First, the results should present answers to each part of the research aim. Second, return to the methods section to ensure that the analysis and variables for each result have been explained.
Begin the results section by describing the number of participants in the final sample and details such as the number who were approached to participate, the proportion who were eligible and who enrolled, and the number of participants who dropped out. The next part of the results should describe the participant characteristics. After that, you may organize your results by the aim or by putting the most exciting results first. Do not forget to report your non-significant associations. These are still findings.
Tables and figures capture the reader’s attention and efficiently communicate your main findings [ 3 ]. Each table and figure should have a clear message and should complement, rather than repeat, the text. Tables and figures should communicate all salient details necessary for a reader to understand the findings without consulting the text. Include information on comparisons and tests, as well as information about the sample and timing of the study in the title, legend, or in a footnote. Note that figures are often more visually interesting than tables, so if it is feasible to make a figure, make a figure. To avoid confusing the reader, either avoid abbreviations in tables and figures, or define them in a footnote. Note that there should not be citations in the results section and you should not interpret results here. Table Table3 3 provides common results section pitfalls and recommendations for addressing them.
Common results section pitfalls and recommendations
Pitfall | Recommendation |
---|---|
The text focuses on statistical tests rather than associations. | The relationships between independent and dependent variables are at the heart of scientific studies and statistical tests are a set of strategies used to elucidate such relationships. For example, instead of reporting that “the odds ratio is 3.4,” report that “women with exposure X were 3.4 times more likely to have disease Y.” There are several ways to express such associations, but all successful approaches focus on the relationships between the variables. |
Causal words like “cause” and “impact” are used inappropriately | Only some study designs and analytic approaches enable researchers to make causal claims. Before you use the word “cause,” consider whether this is justified given your design. Words like “associated” or “related” may be more appropriate. |
The direction of association unclear. |
Instead of “X is associated with Y,” say “an increase in variable X is associated with a decrease in variable Y,” a sentence which more fully describes the relationship between the two variables. |
Discussion Section
Opposite the introduction section, the discussion should take the form of a right-side-up triangle beginning with interpretation of your results and moving to general implications (Fig. 2 ). This section typically begins with a restatement of the main findings, which can usually be accomplished with a few carefully-crafted sentences.

Major elements of the discussion section of an original research article. Often, the elements overlap
Next, interpret the meaning or explain the significance of your results, lifting the reader’s gaze from the study’s specific findings to more general applications. Then, compare these study findings with other research. Are these findings in agreement or disagreement with those from other studies? Does this study impart additional nuance to well-accepted theories? Situate your findings within the broader context of scientific literature, then explain the pathways or mechanisms that might give rise to, or explain, the results.
Journals vary in their approach to strengths and limitations sections: some are embedded paragraphs within the discussion section, while some mandate separate section headings. Keep in mind that every study has strengths and limitations. Candidly reporting yours helps readers to correctly interpret your research findings.
The next element of the discussion is a summary of the potential impacts and applications of the research. Should these results be used to optimally design an intervention? Does the work have implications for clinical protocols or public policy? These considerations will help the reader to further grasp the possible impacts of the presented work.
Finally, the discussion should conclude with specific suggestions for future work. Here, you have an opportunity to illuminate specific gaps in the literature that compel further study. Avoid the phrase “future research is necessary” because the recommendation is too general to be helpful to readers. Instead, provide substantive and specific recommendations for future studies. Table Table4 4 provides common discussion section pitfalls and recommendations for addressing them.
Common discussion section pitfalls and recommendations
Pitfall | Recommendation |
---|---|
The author repeats detailed results or presents new results in the discussion section. | Recall from Fig. that the discussion section should take the shape of a triangle as it moves from a specific restatement of the main findings to a broader discussion of the scientific literature and implications of the study. Specific values should not be repeated in the discussion. It is also not appropriate to include new results in the discussion section. |
The author fails to describe the implication of the study’s limitations. | No matter how well-conducted and thoughtful, all studies have limitations. Candidly describe how the limitations affect the application of the findings. |
Statements about future research are too generic. | Is the relationship between exposure and outcome not well-described in a population that is severely impacted? Or might there be another variable that modifies the relationship between exposure and outcome? This is your opportunity to suggest areas requiring further study in your field, steering scientific inquiry toward the most meaningful questions. |
Follow the Journal’s Author Guidelines
After you select a target journal, identify the journal’s author guidelines to guide the formatting of your manuscript and references. Author guidelines will often (but not always) include instructions for titles, cover letters, and other components of a manuscript submission. Read the guidelines carefully. If you do not follow the guidelines, your article will be sent back to you.
Finally, do not submit your paper to more than one journal at a time. Even if this is not explicitly stated in the author guidelines of your target journal, it is considered inappropriate and unprofessional.
Your title should invite readers to continue reading beyond the first page [ 4 , 5 ]. It should be informative and interesting. Consider describing the independent and dependent variables, the population and setting, the study design, the timing, and even the main result in your title. Because the focus of the paper can change as you write and revise, we recommend you wait until you have finished writing your paper before composing the title.
Be sure that the title is useful for potential readers searching for your topic. The keywords you select should complement those in your title to maximize the likelihood that a researcher will find your paper through a database search. Avoid using abbreviations in your title unless they are very well known, such as SNP, because it is more likely that someone will use a complete word rather than an abbreviation as a search term to help readers find your paper.
After you have written a complete draft, use the checklist (Fig. (Fig.3) 3 ) below to guide your revisions and editing. Additional resources are available on writing the abstract and citing references [ 5 ]. When you feel that your work is ready, ask a trusted colleague or two to read the work and provide informal feedback. The box below provides a checklist that summarizes the key points offered in this article.

Checklist for manuscript quality
(PDF 362 kb)
Acknowledgments
Ella August is grateful to the Sustainable Sciences Institute for mentoring her in training researchers on writing and publishing their research.
Code Availability
Not applicable.
Data Availability
Compliance with ethical standards.
The authors declare that they have no conflict of interest.
Publisher’s Note
Springer Nature remains neutral with regard to jurisdictional claims in published maps and institutional affiliations.
- PRO Courses Guides New Tech Help Pro Expert Videos About wikiHow Pro Upgrade Sign In
- EDIT Edit this Article
- EXPLORE Tech Help Pro About Us Random Article Quizzes Request a New Article Community Dashboard This Or That Game Happiness Hub Popular Categories Arts and Entertainment Artwork Books Movies Computers and Electronics Computers Phone Skills Technology Hacks Health Men's Health Mental Health Women's Health Relationships Dating Love Relationship Issues Hobbies and Crafts Crafts Drawing Games Education & Communication Communication Skills Personal Development Studying Personal Care and Style Fashion Hair Care Personal Hygiene Youth Personal Care School Stuff Dating All Categories Arts and Entertainment Finance and Business Home and Garden Relationship Quizzes Cars & Other Vehicles Food and Entertaining Personal Care and Style Sports and Fitness Computers and Electronics Health Pets and Animals Travel Education & Communication Hobbies and Crafts Philosophy and Religion Work World Family Life Holidays and Traditions Relationships Youth
- Browse Articles
- Learn Something New
- Quizzes Hot
- Happiness Hub
- This Or That Game
- Train Your Brain
- Explore More
- Support wikiHow
- About wikiHow
- Log in / Sign up
- Education and Communications
- College University and Postgraduate
- Academic Writing
- Research Papers
How to Write and Publish Your Research in a Journal
Last Updated: May 26, 2024 Fact Checked
Choosing a Journal
Writing the research paper, editing & revising your paper, submitting your paper, navigating the peer review process, research paper help.
This article was co-authored by Matthew Snipp, PhD and by wikiHow staff writer, Cheyenne Main . C. Matthew Snipp is the Burnet C. and Mildred Finley Wohlford Professor of Humanities and Sciences in the Department of Sociology at Stanford University. He is also the Director for the Institute for Research in the Social Science’s Secure Data Center. He has been a Research Fellow at the U.S. Bureau of the Census and a Fellow at the Center for Advanced Study in the Behavioral Sciences. He has published 3 books and over 70 articles and book chapters on demography, economic development, poverty and unemployment. He is also currently serving on the National Institute of Child Health and Development’s Population Science Subcommittee. He holds a Ph.D. in Sociology from the University of Wisconsin—Madison. There are 13 references cited in this article, which can be found at the bottom of the page. This article has been fact-checked, ensuring the accuracy of any cited facts and confirming the authority of its sources. This article has been viewed 704,617 times.
Publishing a research paper in a peer-reviewed journal allows you to network with other scholars, get your name and work into circulation, and further refine your ideas and research. Before submitting your paper, make sure it reflects all the work you’ve done and have several people read over it and make comments. Keep reading to learn how you can choose a journal, prepare your work for publication, submit it, and revise it after you get a response back.
Things You Should Know
- Create a list of journals you’d like to publish your work in and choose one that best aligns with your topic and your desired audience.
- Prepare your manuscript using the journal’s requirements and ask at least 2 professors or supervisors to review your paper.
- Write a cover letter that “sells” your manuscript, says how your research adds to your field and explains why you chose the specific journal you’re submitting to.

- Ask your professors or supervisors for well-respected journals that they’ve had good experiences publishing with and that they read regularly.
- Many journals also only accept specific formats, so by choosing a journal before you start, you can write your article to their specifications and increase your chances of being accepted.
- If you’ve already written a paper you’d like to publish, consider whether your research directly relates to a hot topic or area of research in the journals you’re looking into.

- Review the journal’s peer review policies and submission process to see if you’re comfortable creating or adjusting your work according to their standards.
- Open-access journals can increase your readership because anyone can access them.

- Scientific research papers: Instead of a “thesis,” you might write a “research objective” instead. This is where you state the purpose of your research.
- “This paper explores how George Washington’s experiences as a young officer may have shaped his views during difficult circumstances as a commanding officer.”
- “This paper contends that George Washington’s experiences as a young officer on the 1750s Pennsylvania frontier directly impacted his relationship with his Continental Army troops during the harsh winter at Valley Forge.”

- Scientific research papers: Include a “materials and methods” section with the step-by-step process you followed and the materials you used. [5] X Research source
- Read other research papers in your field to see how they’re written. Their format, writing style, subject matter, and vocabulary can help guide your own paper. [6] X Research source

- If you’re writing about George Washington’s experiences as a young officer, you might emphasize how this research changes our perspective of the first president of the U.S.
- Link this section to your thesis or research objective.
- If you’re writing a paper about ADHD, you might discuss other applications for your research.

- Scientific research papers: You might include your research and/or analytical methods, your main findings or results, and the significance or implications of your research.
- Try to get as many people as you can to read over your abstract and provide feedback before you submit your paper to a journal.

- They might also provide templates to help you structure your manuscript according to their specific guidelines. [11] X Research source

- Not all journal reviewers will be experts on your specific topic, so a non-expert “outsider’s perspective” can be valuable.

- If you have a paper on the purification of wastewater with fungi, you might use both the words “fungi” and “mushrooms.”
- Use software like iThenticate, Turnitin, or PlagScan to check for similarities between the submitted article and published material available online. [15] X Research source

- Header: Address the editor who will be reviewing your manuscript by their name, include the date of submission, and the journal you are submitting to.
- First paragraph: Include the title of your manuscript, the type of paper it is (like review, research, or case study), and the research question you wanted to answer and why.
- Second paragraph: Explain what was done in your research, your main findings, and why they are significant to your field.
- Third paragraph: Explain why the journal’s readers would be interested in your work and why your results are important to your field.
- Conclusion: State the author(s) and any journal requirements that your work complies with (like ethical standards”).
- “We confirm that this manuscript has not been published elsewhere and is not under consideration by another journal.”
- “All authors have approved the manuscript and agree with its submission to [insert the name of the target journal].”

- Submit your article to only one journal at a time.
- When submitting online, use your university email account. This connects you with a scholarly institution, which can add credibility to your work.

- Accept: Only minor adjustments are needed, based on the provided feedback by the reviewers. A first submission will rarely be accepted without any changes needed.
- Revise and Resubmit: Changes are needed before publication can be considered, but the journal is still very interested in your work.
- Reject and Resubmit: Extensive revisions are needed. Your work may not be acceptable for this journal, but they might also accept it if significant changes are made.
- Reject: The paper isn’t and won’t be suitable for this publication, but that doesn’t mean it might not work for another journal.

- Try organizing the reviewer comments by how easy it is to address them. That way, you can break your revisions down into more manageable parts.
- If you disagree with a comment made by a reviewer, try to provide an evidence-based explanation when you resubmit your paper.

- If you’re resubmitting your paper to the same journal, include a point-by-point response paper that talks about how you addressed all of the reviewers’ comments in your revision. [22] X Research source
- If you’re not sure which journal to submit to next, you might be able to ask the journal editor which publications they recommend.

Expert Q&A
You might also like.

- If reviewers suspect that your submitted manuscript plagiarizes another work, they may refer to a Committee on Publication Ethics (COPE) flowchart to see how to move forward. [23] X Research source Thanks Helpful 0 Not Helpful 0

- ↑ https://www.wiley.com/en-us/network/publishing/research-publishing/choosing-a-journal/6-steps-to-choosing-the-right-journal-for-your-research-infographic
- ↑ https://link.springer.com/article/10.1007/s13187-020-01751-z
- ↑ https://libguides.unomaha.edu/c.php?g=100510&p=651627
- ↑ https://www.canberra.edu.au/library/start-your-research/research_help/publishing-research
- ↑ https://writingcenter.fas.harvard.edu/conclusions
- ↑ https://writing.wisc.edu/handbook/assignments/writing-an-abstract-for-your-research-paper/
- ↑ https://www.springer.com/gp/authors-editors/book-authors-editors/your-publication-journey/manuscript-preparation
- ↑ https://apus.libanswers.com/writing/faq/2391
- ↑ https://academicguides.waldenu.edu/library/keyword/search-strategy
- ↑ https://ifis.libguides.com/journal-publishing-guide/submitting-your-paper
- ↑ https://www.springer.com/kr/authors-editors/authorandreviewertutorials/submitting-to-a-journal-and-peer-review/cover-letters/10285574
- ↑ https://www.apa.org/monitor/sep02/publish.aspx
- ↑ Matthew Snipp, PhD. Research Fellow, U.S. Bureau of the Census. Expert Interview. 26 March 2020.
About This Article

To publish a research paper, ask a colleague or professor to review your paper and give you feedback. Once you've revised your work, familiarize yourself with different academic journals so that you can choose the publication that best suits your paper. Make sure to look at the "Author's Guide" so you can format your paper according to the guidelines for that publication. Then, submit your paper and don't get discouraged if it is not accepted right away. You may need to revise your paper and try again. To learn about the different responses you might get from journals, see our reviewer's explanation below. Did this summary help you? Yes No
- Send fan mail to authors
Reader Success Stories

RAMDEV GOHIL
Oct 16, 2017
Did this article help you?

David Okandeji
Oct 23, 2019

Revati Joshi
Feb 13, 2017

Shahzad Khan
Jul 1, 2017

Apr 7, 2017

Featured Articles

Trending Articles

Watch Articles

- Terms of Use
- Privacy Policy
- Do Not Sell or Share My Info
- Not Selling Info
Don’t miss out! Sign up for
wikiHow’s newsletter

Little-known secrets for how to get published
Advice from seasoned psychologists for those seeking to publish in a journal for the first time
By Rebecca A. Clay
January 2019, Vol 50, No. 1
Print version: page 64

- Peer Review
An academic who is trying to get a journal article published is a lot like a salmon swimming upstream, says Dana S. Dunn, PhD, a member of APA’s Board of Educational Affairs. “The most important thing is persistence,” says Dunn, a psychology professor at Moravian College in Bethlehem, Pennsylvania.
But there are ways to make the journey through the publication process (see The publication process ) easier. “The more work you do up front, the more you can ensure a good outcome,” says Dunn. Among other tasks, that means finding the right venue, crafting the best possible manuscript and not giving up when asked to revise a manuscript.
The Monitor spoke with Dunn and several other senior faculty members with extensive experience publishing articles and serving as journal editors and editorial board members. Here’s their advice.
■ Target the right journals. To find the journal that’s the best fit for your article, research the journals themselves. Check each target journal’s mission statement, ask colleagues who have published there if your work is appropriate for it and read a current issue to see the kinds of articles it contains. “If your work isn’t in line with what they publish, they will reject it out of hand and you will have wasted valuable time,” says Dunn.
Also examine the composition of a journal’s editorial board, which will offer valuable clues about the kind of work the journal values—qualitative versus quantitative research, for example, or single-experiment studies versus multiple-experiment ones. You can even shoot a short email to the editor briefly summarizing your manuscript and asking if it sounds like something he or she feels would be appropriate for the journal. “Editors are pretty good at saying yes or no,” says Dunn.
In addition, let more experienced colleagues assess the strength of your study and give you some ideas about possible venues, says Jerry Suls, PhD, a professor emeritus of psychological and brain sciences at the University of Iowa in Iowa City. Ask how strong and how novel they think your results are and whether your study has any methodological limitations. Although your colleagues may not have a completely accurate view of what journals will and won’t publish, says Suls, it’s still a good idea to get a sense of what they think about your chances.
■ Balance ambition and realism. Aim high, but be realistic about where you send your manuscript. But don’t get too hung up on trying to figure out a hierarchy of which is the “best” journal, says Rose Sokol-Chang, PhD, publisher of journals at APA. Instead, she suggests, think about what you’re trying to achieve with your article. For example, some journals have a longer history, broader focus and higher impact factor, a measure of how often papers in the journal are cited compared to how much is published in the journal. For more narrowly focused research, there are journals focused on subdisciplines that are well-respected by experts and have high impact factors. You could also try highly specialized journals appropriate to your direct area of research, which are more limited in scope and readership. Keep in mind that APA publishes its own journals as well as many affiliated journals, which vary in their levels of specialization.
What you do want to avoid is publishing in one of the increasing number of journals—often online—that aren’t peer-reviewed, says Nova Southeastern University psychology professor Linda Carter Sobell, PhD. Junior faculty may not even be aware that these journals aren’t true academic journals, says Sobell, adding that one possible outcome is that they go up for tenure only to have colleagues point out that their articles are published in nonreputable journals. Tip-offs include nonuniversity addresses or requests that authors pay to publish their work. “You can’t trust them when they say they’re peer-reviewed,” says Sobell. “That could mean the secretary looks at the manuscript when it comes in.”
To check rejection rates, go to www.apa.org/pubs/journals/statistics to get a sense of the odds for APA journals. For other journals, contact the editor, check the publication’s website or directions to contributors or see if your institution subscribes to the Cabells database, which offers information on both reputable journals and those it deems “predatory.”
Also, be sure to submit your work to just one journal at a time. Shotgunning a manuscript to multiple outlets simultaneously “is completely verboten,” says Suls.
■ Hone your manuscript. Give yourself time to write the best manuscript you can, says APA Board of Scientific Affairs (BSA) member Keith F. Widaman, PhD, distinguished professor of the Graduate Division of the Graduate School of Education at the University of California, Riverside. To make sure your writing is first-rate, study “The Elements of Style,” says Widaman, who rereads the William Strunk and E.B. White classic every year or two. Learn the difference between active and passive voices, the difference between “that” and “which,” and when to use commas. “There are times when you misportray the meaning of what you’re trying to get across if you write something poorly,” he says.
Before you start writing, draft an outline with subheads that mimic how manuscripts are organized in APA journals. “Carefully constructing a manuscript helps the reader follow your thinking,” he says. Also consult APA’s newly revised Journal Article Reporting Standards (APA Style JARS), which offer guidance on what information needs to be included in a research manuscript, whether your manuscript covers quantitative research or qualitative research ( American Psychologist , Vol. 73, No. 1, 2018), suggests Sokol-Chang.
Be sure to pay close attention to details such as spelling and footnotes, Widaman adds. “If a person does a crummy job with references, for example, what other details are they not paying attention to?” To this end, take advantage of APA Style CENTRAL , which offers manuscript templates, preformatted references drawn from APA’s PsycINFO database and more.
And polish your manuscript by asking colleagues—both in and out of your specialty area—to offer constructive criticism. Make sure you haven’t overlooked relevant citations, which will suggest to reviewers that you don’t know the literature and where your work fits in. Consider having a statistician double-check your analyses.
■ Be prepared to revise. Most manuscripts are going to be rejected, so don’t take rejection personally, says Suls. It’s also extremely rare for a journal to accept a manuscript as is. Of the thousand-plus manuscripts Suls saw as associate editor of the Journal of Personality and Social Psychology, he remembers only two that received a thumbs-up without requests for at least minor revisions. “Most papers are going to be rejected or are going to be resubmitted with revisions,” says Suls. “You’re not the only one.”
Your initial reaction to reviewers’ feedback may be disappointment or even anger. Put the comments aside for a few days while you calm down. “The first thing to realize is that reviewers are not trying to do a search-and-destroy mission; they’re trying to be helpful,” says Dunn. “Often when you read the comments with a colder eye, you realize the comments are good ones that will improve your work.”

For less extreme problems, the editor may invite you to revise the rejected paper and resubmit it or suggest that you send your manuscript elsewhere. Either way, be just as diligent in revising your manuscript as you were in writing it, says Dunn. Thank the reviewers, address every comment they made and use a detailed cover letter to explain those changes, with page numbers so the editor can easily see how you’ve addressed concerns. If reviewers disagree on a particular point and the editor hasn’t chimed in, choose which side you agree with and explain why you chose that side. And if you disagree with a comment and decide to reject that advice, explain that too.
■ Gain experience. Being on the other side of the editorial process can help give you ideas about how to better craft your own manuscripts. If you’re a graduate student or junior faculty member, ask a mentor or colleague who frequently serves as a reviewer if you can become a co-reviewer. “That’s a valuable educational experience,” says Dunn. (See “ How to Review a Manuscript ” in the May 2018 Monitor for more insights.)
If you do get a chance to review someone else’s manuscript, do it well and turn it in quickly. “If it comes back in a very timely fashion and the review is a good one, that person will be used again,” says Suls. That said, junior faculty should make sure the review process does not cut into the time they devote to doing their own research and writing.
■ Keep trying. Finally, don’t give up if your article is rejected by the first publication you send it to, says BSA member Jeffrey M. Zacks, PhD, a professor of psychological and brain sciences and radiology at Washington University in St. Louis. As long as your research is fundamentally sound, says Zacks, “there’s usually another reasonable journal you can turn around and go to.”
For a set of interactive modules on publishing journal articles, reviewing journal manuscripts and other issues of importance to early career academics and researchers, see APA’s Science Career Series at www.apa.org/career-development/courses .
Further reading
Managing Your Research Data and Documentation Berenson, K.R. APA, 2017
How to Publish High-Quality Research Joireman, J., & Van Lange, P.A.M. APA, 2015
Write It Up: Practical Strategies for Writing and Publishing Journal Articles Silvia, P.J. APA, 2015
Related Articles
- The publication process
Key takeaways
1: Find the right journal for your research.
2: Write carefully and double-check your analyses.
3: Be open to revisions.
4: Don’t give up after a rejection—rework and resubmit.
Letters to the Editor
- Send us a letter
- Advanced search
- Peer review

Discover relevant research today

Advance your research field in the open

Reach new audiences and maximize your readership
ScienceOpen puts your research in the context of
Publications
For Publishers
ScienceOpen offers content hosting, context building and marketing services for publishers. See our tailored offerings
- For academic publishers to promote journals and interdisciplinary collections
- For open access journals to host journal content in an interactive environment
- For university library publishing to develop new open access paradigms for their scholars
- For scholarly societies to promote content with interactive features
For Institutions
ScienceOpen offers state-of-the-art technology and a range of solutions and services
- For faculties and research groups to promote and share your work
- For research institutes to build up your own branding for OA publications
- For funders to develop new open access publishing paradigms
- For university libraries to create an independent OA publishing environment
For Researchers
Make an impact and build your research profile in the open with ScienceOpen
- Search and discover relevant research in over 95 million Open Access articles and article records
- Share your expertise and get credit by publicly reviewing any article
- Publish your poster or preprint and track usage and impact with article- and author-level metrics
- Create a topical Collection to advance your research field

Create a Journal powered by ScienceOpen
Launching a new open access journal or an open access press? ScienceOpen now provides full end-to-end open access publishing solutions – embedded within our smart interactive discovery environment. A modular approach allows open access publishers to pick and choose among a range of services and design the platform that fits their goals and budget.
Continue reading “Create a Journal powered by ScienceOpen”
What can a Researcher do on ScienceOpen?
ScienceOpen provides researchers with a wide range of tools to support their research – all for free. Here is a short checklist to make sure you are getting the most of the technological infrastructure and content that we have to offer. What can a researcher do on ScienceOpen? Continue reading “What can a Researcher do on ScienceOpen?”
ScienceOpen on the Road
Upcoming events.
- 15 June – Scheduled Server Maintenance, 13:00 – 01:00 CEST
Past Events
- 20 – 22 February – ResearcherToReader Conference
- 09 November – Webinar for the Discoverability of African Research
- 26 – 27 October – Attending the Workshop on Open Citations and Open Scholarly Metadata
- 18 – 22 October – ScienceOpen at Frankfurt Book Fair.
- 27 – 29 September – Attending OA Tage, Berlin .
- 25 – 27 September – ScienceOpen at Open Science Fair
- 19 – 21 September – OASPA 2023 Annual Conference .
- 22 – 24 May – ScienceOpen sponsoring Pint of Science, Berlin.
- 16-17 May – ScienceOpen at 3rd AEUP Conference.
- 20 – 21 April – ScienceOpen attending Scaling Small: Community-Owned Futures for Open Access Books .
What is ScienceOpen?
- Smart search and discovery within an interactive interface
- Researcher promotion and ORCID integration
- Open evaluation with article reviews and Collections
- Business model based on providing services to publishers
Live Twitter stream
Some of our partners:.

View the latest institution tables
View the latest country/territory tables
These 10 institutions published the most papers in Nature and Science in 2018
From CRISPR to CLARITY, here are some of the most high-profile studies.
Gemma Conroy, Bec Crew
Gene-editing tech, CRISPR-Cas9, featured in one of Harvard's most widely discussed Nature papers in 2017. Credit: Meletios Verras/Getty Images
3 September 2019
Meletios Verras/Getty Images
Gene-editing tech, CRISPR-Cas9, featured in one of Harvard's most widely discussed Nature papers in 2017.
The journals Nature and Science are where some of the highest quality research is showcased to the world.
The institutions listed below were the largest contributors to papers published in Nature and Science in 2018, as tracked by the Nature Index.
View the Nature Index 2019 Annual Tables for Nature and Science .
1. Harvard University
Fractional count: 70.67 (-15.3%), Article count: 210
No research institute beats Harvard University for papers published in Nature and Science .
As one of the oldest universities in the United States , and one of the world’s leading higher education institutions, Harvard has a storied history of revolutionary discoveries, including the smallpox vaccine, anaesthesia, and oral contraception.
More recently, widely discussed studies by Harvard scientists include a 2017 Nature paper that described a method for storing digital data in bacterial genomes using CRISPR-Cas9 gene-editing technology, and a Science paper this year , in which researchers from Harvard’s Institute for Quantitative Social Science analyzed the effect of fake news on Twitter during the 2016 US presidential election.
2. Stanford University
Fractional Count: 39.85 (-24.3%); Article Count: 100
Stanford University appears in the upper echelons of many of the Nature Index Annual Tables rankings, including chemistry, life sciences, the physical sciences, and academic institutions. It’s also the second most prolific publisher in Nature and Science .
Top authors at Stanford include bioengineer and neuroscientist, Karl Deisseroth , whose 2013 Nature paper on a new technology named CLARITY describes how mammalian brains can be rendered “clear as Jell-O” for access by molecular probes, as The New York Times put it .
Computer scientist, Fei-Fei Li, co-director of Stanford's Human-Centered AI Institute and Vision and Learning Lab, is one of the most prolific researchers in the field of AI, and has published on machine learning, deep learning, computer vision and cognitive neuroscience.
3. Massachusetts Institute of Technology
Fractional count: 37.69 (13.3%), Article count: 130
As one of the world’s most prestigious higher education institutions, MIT has been at the frontier of research for more than 150 years, its close ties with industry fostering an emphasis on entrepreneurship and applied science.
With 12,707 faculty and staff on campus and an annual budget of more than US$3.5 billion (2018), almost 85% of MIT’s undergraduates engage in frontline, faculty-led research.
One of MIT’s most talked-about studies in 2018 was a Science paper that analysed the spread of true and false news. Garnering an Altmetrics score of more than 9,600, which includes more than 8,000 tweets and 360 news stories, it lent support to the adage: lies spread faster than the truth.
A 2018 Nature paper by MIT researchers on the ‘Moral Machine experiment’ also drew significant attention from the wider public, posing complex questions about how AI in future will make moral decisions.
4. Max Planck Society
Fractional Count: 32.35 (-15%) Article count: 139
From nuclear fission to the theory of relativity, Germany's Max Planck Society has been the birthplace of some of the most important discoveries in science.
Among Max Planck’s Nature and Science findings published last year were a new antiretroviral treatment for HIV , graphene nanoribbons , and a new method for capturing changes in RNA .
One of Max Planck’s most highly cited Nature papers of 2018 presented the most precise measurements of the electron ever made. The findings support the standard model of particle physics, which proposes that electrons maintain a near perfect spherical shape.
Along with an international team, Max Planck researchers published a paper in Science last year that revealed the source of cosmic neutrinos, a type of high-energy particle that travels over vast distances. The study found that neutrinos originate from blazars, a type of galaxy powered by supermassive black holes.
5. University of California, Berkeley
Fractional count: 24.30 (39.4%), Article count: 78
Credited for the discovery or co-discovery of 16 elements — more than any other university — the University of California, Berkeley (UC Berkeley) has a well-established history of scientific advances. You can see its fingerprints on 97 berkelium (Bk), 98 californium (Cf) and 95 americium (Am).
Among its most highly-cited Nature and Science papers of last year were reports on the genetic basis of psychiatric disorders ; a possible cause of fast radio bursts ; and cobalt-free batteries .
In February 2018 , UC Berkeley researchers were involved in the development of genome-editing tool that can detect human papillomavirus with great sensitivity. The study was the institution’s most highly-cited Science paper of that year.
A paper published in Nature also attracted a lot of attention in 2018, when UC Berkeley researchers, along with an international team, described a microchip that uses light to transmit data. The new device is faster and more efficient than conventional silicon chip technologies.
6. University of California, Los Angeles
Fractional Count: 21.11 (82.1%), Article count: 67
Having just celebrated its 100th birthday, the University of California, Los Angeles (UCLA) is one of the youngest universities in Nature Index’s top 10 tables, and yet it receives the most student applications of any university in the US.
Key papers published in Science last year include a study that revealed how certain psychiatric disorders share global gene expression patterns, and one describing how UCLA engineers 3D-printed an AI device that identifies objects at the speed of light, a promising development for medicine, robotics and security .
As vice-chancellor for research, Roger Wakimoto, says, UCLA’s growth and achievements mirror the trajectory of the city it calls home.
“I believe the institution’s success is based on its strong commitment to teaching, recruiting and retaining some of the most talented researchers in the world, and having a close and synergistic relationship with the city of Los Angeles.”
7. Yale University
Fractional count: 19.75 (36.8%), Article count: 57
From finding evidence of time crystals to discovering how gut bacteria cause autoimmune disease, Yale University’s research consistently breaks ground. As one of the oldest universities in the US, it’s also built a strong record of highly-cited papers published in the world’s leading scientific journals.
Last year, Yale astronomers made headlines when they discovered that the galaxy NGC 1052-DF2 contained almost no dark matter, challenging the assumption that it’s essential for the formation of galaxies. The study was one of Yale’s most talked-about Nature papers of 2018.
A Science study involving Yale researchers on how household products contribute to air pollution also sparked conversation last year. The team found that cleaning products, perfumes and shampoos rival transport as major emissions sources.
8. Columbia University in the City of New York
Fractional count: 19.67 (-4.3%), Article count: 65
Established 265 years ago by royal charter of King George II of England, Columbia University is the oldest institution of higher learning in New York City and the fifth oldest in the US.
With more than 200 research centres and institutes, 350 new inventions each year and 84 Nobel laureates, Columbia is at the forefront of scientific discovery.
In 2018, Columbia University produced 65 papers in Nature and Science , placing it in eighth place in this ranking. The institution’s research performance is particularly strong in the life sciences, which accounts for around half of its overall output.
Last year, researchers from Columbia co-authored a study suggesting that 60% of people with European descent living in the US can be genetically identified using available genetic information, even if they have not undergone genetic testing.
Published in Science , the findings highlight the need for strategies that safeguard genetic privacy.
9. University of Oxford
Fractional count: 19.56 (73.6%), Article count: 73
The University of Oxford is the oldest in the English-speaking world, with teaching dating back as far as 1096. Today, it maintains its prestigious reputation with eighth place among the leading academic institutions in the Nature Index.
The largest share of Oxford’s scientific output is in the life sciences, with papers in the discipline contributing around one-third of its output.
Research funding provided by councils, trusts and industry is Oxford’s largest source of income, accounting for 26% of its funds — the highest research income of all the United Kingdom's universities.
Oxford is also home to the Beecroft Building, a new US$64-million facility dedicated to experimental and theoretical physics with laboratories designed for making precise measurements at the atomic level.
In 2018, Oxford researchers co-authored an influential analysis of how to reduce the environmental impacts of food. The paper, published in Science , triggered widespread debate about food choices, such as avoiding dairy and meat.
10. Swiss Federal Institute of Technology Zurich
Fractional count: 19.23 (29.1%), Article count: 55
Boasting 21 Nobel Prize winners, two Fields medallists and a Turing Award recipient, the Swiss Federal Institute of Technology Zurich (ETH Zurich) has built a reputation for trailblazing research over the past 160 years.
The technical and scientific university is also renowned for its ability to produce highly-cited research, taking tenth place among the top institutions in Nature and Science papers.
Among the most talked-about papers from the university are predictions of increased marine heatwaves under climate change; a technique for transmitting quantum information ; and detailed images of proteins involved in opioid signalling.
In January 2018 , researchers at ETH Zurich discovered how a particular group of nanocrystals are able to emit a bright light. The study, which was the most highly-cited Nature article from the institution that year, could have a range of applications in materials science, from data transmission to supercomputers.
The top 10 academic institutions in 2018: normalized
The top 10 academic institutions in 2018
The top 10 countries for scientific research in 2018
Correction: Text amended to remove "in the 21 century" from the opening sentence of the Max Planck Society's profile.
Sign up to the Nature Index newsletter
Get regular news, analysis and data insights from the editorial team delivered to your inbox.
- Sign up to receive the Nature Index newsletter. I agree my information will be processed in accordance with the Nature and Springer Nature Limited Privacy Policy .
Research Papers - Science topic

- Recruit researchers
- Join for free
- Login Email Tip: Most researchers use their institutional email address as their ResearchGate login Password Forgot password? Keep me logged in Log in or Continue with Google Welcome back! Please log in. Email · Hint Tip: Most researchers use their institutional email address as their ResearchGate login Password Forgot password? Keep me logged in Log in or Continue with Google No account? Sign up
share this!
April 27, 2022
Millions of research papers are published in a year. How do scientists keep up?
by Eva Botkin-Kowacki, Northeastern University
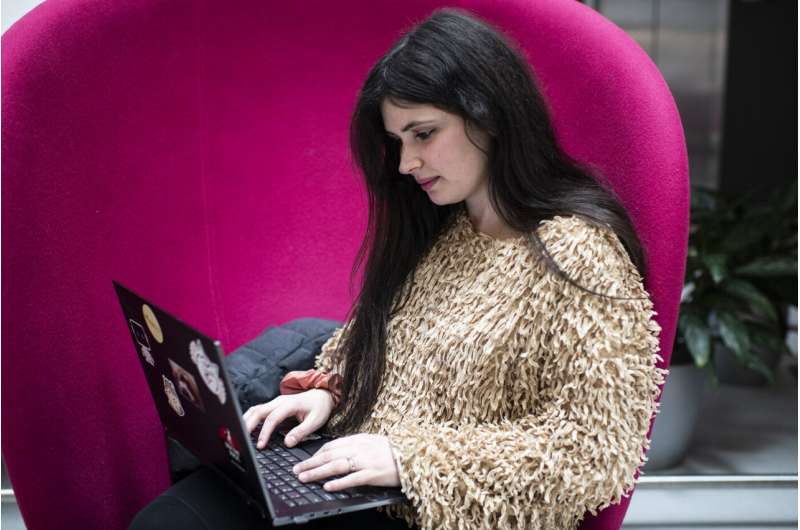
If you want to be a scientist, you're going to have to do a lot of reading.
Science is an endeavor focused on building and sharing knowledge. Researchers publish papers detailing their discoveries, breakthroughs, and innovations in order to share those revelations with colleagues. And there are millions of scientific papers each year.
Keeping up with the latest developments in their field is a challenge for researchers at all points of their careers, but it especially affects early-career scientists, as they also have to read the many papers that represent the foundation of their field.
"It's impossible to read everything. Absolutely impossible," Ajay Satpute, director of the Affective and Brain Science Lab and an assistant professor of psychology at Northeastern. "And if you don't know everything that has happened in the field, there's a real chance of reinventing the wheel over and over and over again." The challenge, he says, is to figure out how to train the next generation of scientists economically, balancing the need to read all the seminal papers with training them as researchers in their own right.
That task is only getting more difficult, says Alessia Iancarelli, a Ph.D student studying affective and social psychology in Satpute's lab. "The volume of published literature just keeps increasing," she says. "How are scientists able to develop their scholarship in a field given this huge amount of literature?" They have to pick and choose what to read.
But common approaches to that prioritization, Iancarelli says, can incorporate biases and leave out crucial corners of the field. So Iancarelli, Satpute and colleagues developed a machine learning approach to find a better—and less biased—way to make a reading list. Their results, which were published last week in the journal PLOS One , also help reduce gender bias.
"There really is a problem about how we develop scholarship," Satpute says. Right now, scientists will often use a search tool like Google Scholar on a topic and start from there, he says. "Or, if you're lucky, you'll get a wonderful instructor and have a great syllabus. But that's going to be basically the field through that person's eyes. And so I think that this really fills a niche that might help create balance and cross-disciplinary scholarship without necessarily having access to a wonderful instructor, because not everyone gets that."
The problem with something like Google Scholar, Iancarelli explains, is that it will give you the most popular papers in a field, measured by how many other papers have cited them. If there are subsets of that field that aren't as popular but are still relevant, the important papers on those topics might get missed with such a search.
Take, for example, the topic of aggression (which is the subject the researchers focused on to develop their algorithm). Media and video games are a particularly hot topic in aggression research, Iancarelli says, and therefore there are a lot more papers on that subset of the field than on other topics, such as the role of testosterone, and social aggression.
So Iancarelli decided to group papers on the topic of aggression into communities. Using citation network analysis, she identified 15 research communities on aggression. Rather than looking at the raw number of times a paper has been cited in another research paper , the algorithm determines a community of papers that tend to cite each other or the same core set of papers. The largest communities it revealed were media and video games, stress, traits and aggression, rumination and displaced aggression, the role of testosterone, and social aggression. But there were also some surprises, such as a smaller community of research papers focused on aggression and horses.
"If you use community detection, then you get this really rich, granular look at the aggression field," Satpute says. "You have sort of a bird's-eye-view of the entire field rather than [it appearing that] the field of aggression is basically media, video games, and violence."
In addition to diversifying the topics featured by using this community approach, the researchers also found that the percentage of articles with women first authors dubbed influential by the algorithm doubled in comparison to when they focused only on total citation counts. (Iancarelli adds there might be some biases baked into that result, as the team couldn't ask the authors directly about their gender identity and instead had to rely on assumptions based on the author's name, picture, and any pronouns used to refer to them.)
The team has released the code behind this algorithm so that others can use it and replicate their citation network analysis approach in other fields of research.
For Iancarelli, there's another motivation: "I would love to use this work to create a syllabus and teach my own course on human aggression. I would really love to base the syllabus on the most relevant papers from each different community to give a true general view of the human aggression field."
Journal information: PLoS ONE
Provided by Northeastern University
Explore further
Feedback to editors

'Screaming Woman' mummy may have died in agony 3,500 years ago
2 hours ago

Physicists report new insights into exotic particles key to magnetism
10 hours ago

A Band-Aid for the heart? New 3D printing method makes this, and much more, possible

Study shows link between asymmetric polar ice sheet evolution and global climate
12 hours ago

Microscopy breakthrough promises better imaging for sensitive materials

Plant biologists discover an ancient gene family is responsible for plant prickles across species

Researchers uncover 500 million-year-old mollusk ancestor

Retreating Andean rocks signal the world's glaciers are melting far faster than predicted, report scientists

When it comes to DNA replication, humans and baker's yeast are more alike than different, scientists discover

Astronomers use AI to find elusive stars 'gobbling up' planets
14 hours ago
Relevant PhysicsForums posts
Bach, bach, and more bach please.
8 hours ago
Cover songs versus the original track, which ones are better?
16 hours ago
Biographies, history, personal accounts
Jul 31, 2024
Imperial War Museum London (And similar organisations globally)
What are your favorite disco "classics", today's fusion music: t square, cassiopeia, rei & kanade sato.
Jul 30, 2024
More from Art, Music, History, and Linguistics
Related Stories

Prize winning topics found to deliver more science papers and citations than non-prize-winning topics
Oct 6, 2021

Women are undercited and men are overcited in communication
Aug 6, 2021

Women more likely to enjoy aggression in porn: study
Feb 11, 2022

Analysis suggests China has passed US on one research measure
Mar 8, 2022

Successful research papers cite young references
Apr 15, 2019

Seattle AI lab's free search engine aims to accelerate scientific breakthroughs
Oct 31, 2019
Recommended for you

Autonomy boosts college student attendance and performance

Study reveals young scientists face career hurdles in interdisciplinary research
Jul 29, 2024

Transforming higher education for minority students: Minor adjustments, major impacts

Saturday Citations: E-bike accident spike; epigenetics in memory formation; Komodo dragons now scarier
Jul 27, 2024

Communicating numbers boosts trust in climate change science, research suggests
Jul 26, 2024

Saturday Citations: Scientists study monkey faces and cat bellies; another intermediate black hole in the Milky Way
Jul 20, 2024
Let us know if there is a problem with our content
Use this form if you have come across a typo, inaccuracy or would like to send an edit request for the content on this page. For general inquiries, please use our contact form . For general feedback, use the public comments section below (please adhere to guidelines ).
Please select the most appropriate category to facilitate processing of your request
Thank you for taking time to provide your feedback to the editors.
Your feedback is important to us. However, we do not guarantee individual replies due to the high volume of messages.
E-mail the story
Your email address is used only to let the recipient know who sent the email. Neither your address nor the recipient's address will be used for any other purpose. The information you enter will appear in your e-mail message and is not retained by Phys.org in any form.
Newsletter sign up
Get weekly and/or daily updates delivered to your inbox. You can unsubscribe at any time and we'll never share your details to third parties.
More information Privacy policy
Donate and enjoy an ad-free experience
We keep our content available to everyone. Consider supporting Science X's mission by getting a premium account.
E-mail newsletter
- Frontiers in Science
- Article hubs
- Imperatives for reducing methane emissions
The methane imperative
Lead article, explore article hub, read article explainer.
- 1 Nicholas School of the Environment, Duke University, Durham, NC, United States
- 2 The Porter School of the Environment and Earth Sciences, Tel Aviv University, Ramat Aviv, Israel
- 3 SRON Netherlands Institute for Space Research, Leiden, Netherlands
- 4 World Energy Outlook Team, International Energy Agency (IEA), Paris, France
- 5 Institute for Governance & Sustainable Development (IGSD), Washington, DC, United States
- 6 Department of Physics, Georgetown University, Washington, DC, United States
- 7 International Institute for Applied Systems Analysis, Laxenburg, Austria
- 8 Earth Sciences Division, NASA Goddard Space Flight Center, Greenbelt, MD, United States
- 9 Laboratoire des Sciences du Climat et de l’Environnement, LSCE-IPSL (CEA-CNRS-UVSQ), Université Paris-Saclay, Gif-sur-Yvette, France
- 10 NASA Goddard Institute for Space Studies, New York, NY, United States
- 11 Laboratoire des Sciences du Climat et de l’Environnement, UMR 8212 CEA-CNRS-UVSQ, Institut Pierre-Simon Laplace, Université de Saclay, Saclay, France
- 12 Global Science, The Nature Conservancy, Arlington, VA, United States
- 13 Department of Marine, Earth and Atmospheric Sciences, North Carolina State University, Raleigh, NC, United States
- 14 Center for Climate Systems Research, Columbia University, New York, NY, United States
Anthropogenic methane (CH 4 ) emissions increases from the period 1850–1900 until 2019 are responsible for around 65% as much warming as carbon dioxide (CO 2 ) has caused to date, and large reductions in methane emissions are required to limit global warming to 1.5°C or 2°C. However, methane emissions have been increasing rapidly since ~2006. This study shows that emissions are expected to continue to increase over the remainder of the 2020s if no greater action is taken and that increases in atmospheric methane are thus far outpacing projected growth rates. This increase has important implications for reaching net zero CO 2 targets: every 50 Mt CH 4 of the sustained large cuts envisioned under low-warming scenarios that are not realized would eliminate about 150 Gt of the remaining CO 2 budget. Targeted methane reductions are therefore a critical component alongside decarbonization to minimize global warming. We describe additional linkages between methane mitigation options and CO 2 , especially via land use, as well as their respective climate impacts and associated metrics. We explain why a net zero target specifically for methane is neither necessary nor plausible. Analyses show where reductions are most feasible at the national and sectoral levels given limited resources, for example, to meet the Global Methane Pledge target, but they also reveal large uncertainties. Despite these uncertainties, many mitigation costs are clearly low relative to real-world financial instruments and very low compared with methane damage estimates, but legally binding regulations and methane pricing are needed to meet climate goals.
- The atmospheric methane growth rates of the 2020s far exceed the latest baseline projections; methane emissions need to drop rapidly (as do CO 2 emissions) to limit global warming to 1.5°C or 2°C.
- The abrupt and rapid increase in methane growth rates in the early 2020s is likely attributable largely to the response of wetlands to warming with additional contributions from fossil fuel use, in both cases implying that anthropogenic emissions must decrease more than expected to reach a given warming goal.
- Rapid reductions in methane emissions this decade are essential to slowing warming in the near future, limiting overshoot by the middle of the century and keeping low-warming carbon budgets within reach.
- Methane and CO 2 mitigation are linked, as land area requirements to reach net zero CO 2 are about 50–100 million ha per GtCO 2 removal via bioenergy with carbon capture and storage or afforestation; reduced pasture is the most common source of land in low-warming scenarios.
- Strong, rapid, and sustained methane emission reduction is part of the broader climate mitigation agenda and complementary to targets for CO 2 and other long-lived greenhouse gases, but a net zero target specifically for methane is neither necessary nor plausible.
- Many mitigation costs are low relative to real-world financial instruments and very low compared with methane damage estimates, but legally binding regulations and widespread pricing are needed to encourage the uptake of even negative cost options.
Introduction
Worldwide efforts to limit climate change are rightly focused on carbon dioxide (CO 2 ), the primary driver ( 1 ). However, since humanity has failed to adequately address climate change for several decades, keeping warming below agreed goals now requires that we address all major climate pollutants. Methane is the second most important greenhouse gas driving climate change. Out of a total observed warming of 1.07°C during the period 2010 to 2019, the Working Group I (WGI) 2021 Intergovernmental Panel on Climate Change (IPCC) Sixth Assessment Report (AR6) attributed 0.5°C to methane emissions ( 1 ). However, in many respects, methane mitigation has been neglected relative to CO 2 . For example, only ~2% of global climate finance is estimated to go towards methane abatement ( 2 ). Similarly, only about 13% of global methane emissions are covered by current policy mechanisms ( 3 ). With dramatic climate changes already occurring and methane providing substantial leverage to slow warming in the near future and reduce surface ozone pollution, political will to mitigate methane has recently increased, especially following the Global Methane Assessment (GMA) published by the United Nations Environment Programme (UNEP) and the Climate and Clean Air Coalition (CCAC) in May 2021 ( 4 ). The Assessment showed that reducing methane was an extremely cost-effective way to rapidly slow warming and contribute to climate stabilization while also providing large benefits to human health, crop yield, and labor productivity. The GMA also demonstrated that various technical and behavioral options were currently available to achieve such emission cuts. Drawing upon that Assessment and related analysis ( 5 ), the United States and European Union launched the Global Methane Pledge (GMP) in November 2021 at the 26th Conference of the Parties to the United Nations Framework Convention on Climate Change (COP26), under which countries set a collective goal of reducing anthropogenic methane emissions by at least 30% (relative to 2020 levels) by 2030. By COP28 in November 2023, participation in the GMP had increased to 155 countries that collectively account for more than half of global anthropogenic methane emissions.
However, far more needs to be done if the world is to change the current methane trajectory and meet the goals of the GMP and other national pledges. This article presents three imperatives supported by a series of analyses (detailed further in Methods):
● Imperative 1—to change course and reverse methane emissions growth—describes changes in methane observed during the recent past and projected for the near future and compares these with low-warming scenarios (Analysis A).
● Imperative 2—to align methane and CO 2 mitigation — discusses methane targets and metrics (Analysis B), investigates the connections between methane emissions and CO 2 mitigation efforts (Analysis C), and assesses their impacts (Analyses D–F).
● Imperative 3—to optimize methane abatement options and policies—presents analyses of the mitigation potential of national-level abatement options (Analysis G) and evaluates their cost-effectiveness (Analysis H) across the 50 countries with greatest mitigation potential by subsector (i.e., landfill, coal, oil, and gas) using a novel tool. We also compare profit versus pricing from controlling methane emissions from oil production (Analysis I) and describe ongoing efforts to support national and regional decision-making.
Finally, we outline paths forward for improving scientific understanding of methane emissions, abatement opportunities, and physical processes that will affect future methane levels in the atmosphere.
Imperative 1—to change course and reverse methane emissions growth
Atmospheric methane concentrations are rising faster than projections.
Scenarios consistent with temperature goals to limit warming to 1.5°C, or well below 2°C, with no or limited overshoot include large and rapid reductions in methane ( 4 , 6 ). In the real world, however, atmospheric methane has been rising rapidly since 2006 and by the end of the 2010s reached 5-year average growth rates not seen since the 1980s ( 4 , 7 , 8 ). Methane concentration increases in 2021 are the largest recorded, with high values throughout the period 2020 to 2023 (Analysis A; Figure 1A ). The uncertainty ranges from the ground-based and satellite datasets typically overlap, leading to high confidence in the growth rate values. Using a mass balance approach assuming that the methane loss rate is proportional to the atmospheric methane loading (i.e., a constant methane atmospheric lifetime of 9.1 yr) ( 12 ), emissions appear to have risen substantially from 2020 to 2023 ( Figure 1B ).
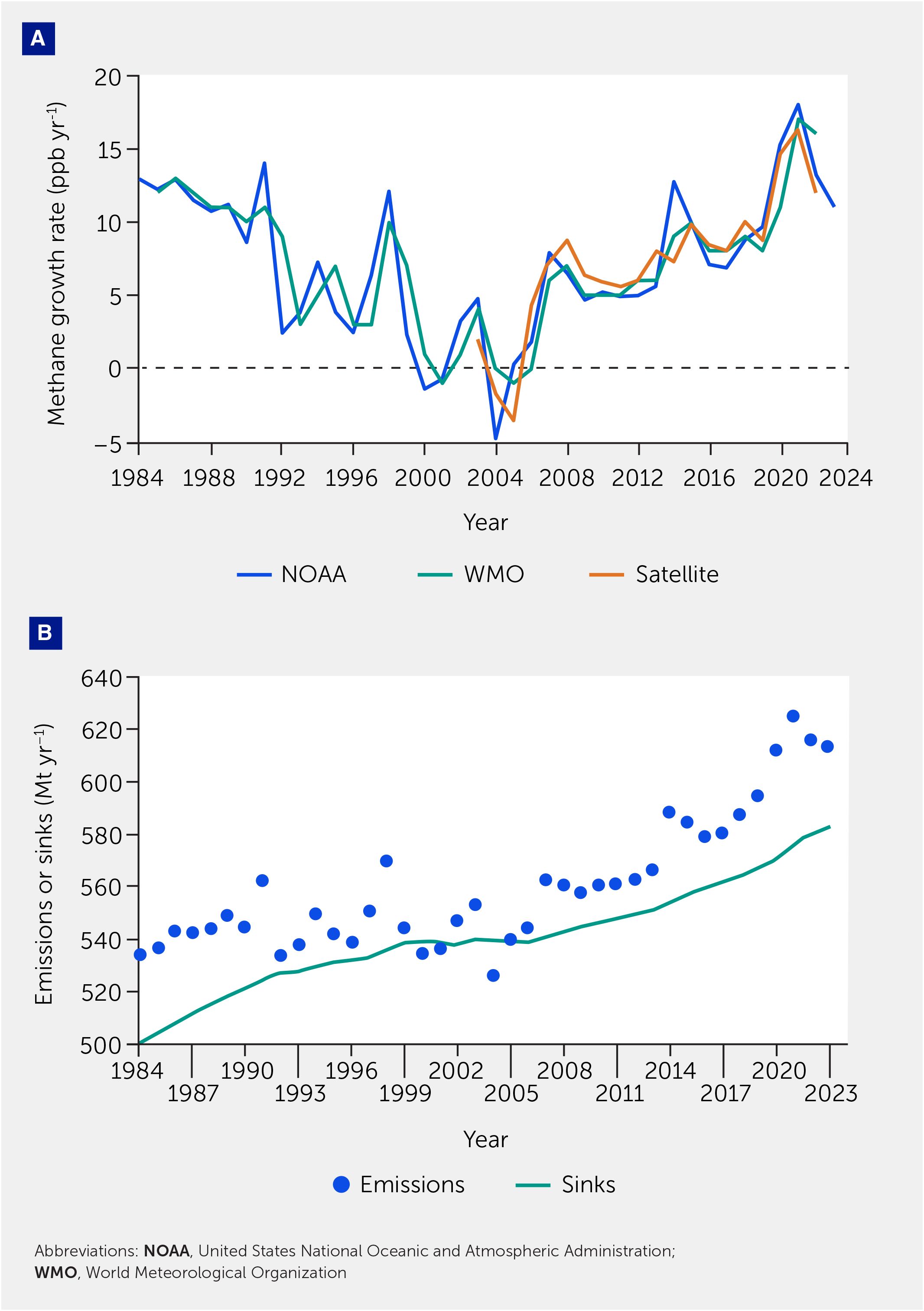
Figure 1 Accelerating methane growth rates and emissions over recent decades. (A) Observed methane annual growth rates (ppb yr −1 ) through 2022 or 2023 from the ground-based networks of the United States National Oceanic and Atmospheric Administration (NOAA) ( 9 ) and the World Meteorological Organization ( 10 ) and from satellite data from the Copernicus Atmospheric Monitoring Service (CAMS) ( 11 ) total column datasets. (B) Estimated emissions and sinks through 2023 based on the NOAA abundance observations. Emissions and sinks estimates are based on a simple box model assuming sinks are proportional to the atmospheric abundance of methane. Uncertainties in the ground-based and satellite data are around 0.5 ppb yr −1 , and 3 ppb yr −1 , respectively. See Methods (Analysis A) for further details. Data for this and other figures are available in Supplementary Table 1 .
We compare the observed atmospheric methane growth rates with values under recent baseline scenarios developed with integrated assessment models (IAMs) in the early 2020s and “bottom-up” engineering approach models. All include data on actual developments through the period ~2018 to 2020 ( 13 ). The observed growth rates are roughly 1.5- to 2.5-fold higher than the multi-model mean baseline or bottom-up projections from 2020 to 2022 ( Figure 2 ). The observed growth rates also exceed any individual model’s baseline projections during that period. Observed 2023 growth rates show the highest values of any individual model, well above multi-model means or bottom-up analyses. Baseline scenarios are used to analyze how additional technical, behavioral, and policy options can mitigate climate change. That real-world methane growth rates exceed baseline projections therefore indicates that policies may have to be even stronger than those in existing analyses to reach the Paris Agreement’s goals. Indeed, comparisons of observed atmospheric growth rates with those in 1.5°C-consistent scenarios (using the 2018 IPCC scenarios that did not include observations past 2017) show enormous differences ( Figure 2 ), emphasizing how much stronger policies need to be to reach low-warming goals.
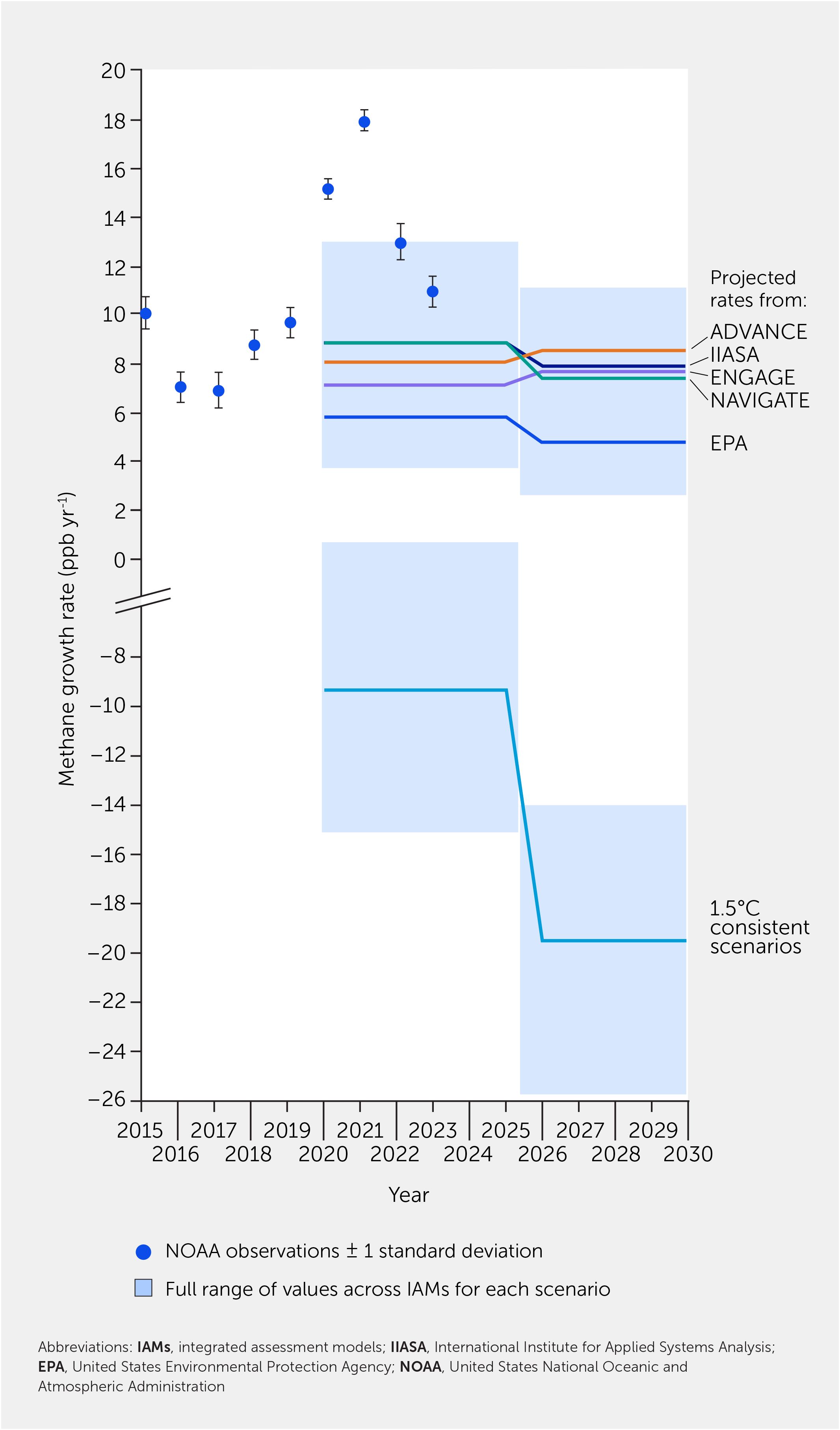
Figure 2 Projected and observed methane growth rates. Methane abundance growth rates during the 2020s from baseline scenarios from the ADVANCE ( https://www.fp7-advance.eu/ ), NAVIGATE ( https://www.navigate-h2020.eu/ ) ( 14 ), and ENGAGE ( https://www.engage-climate.org/ ) projects using integrated assessment models (IAMs; data show multi-model means) and from the “bottom-up” analyses of the International Institute for Applied Systems Analysis (IIASA) ( 15 ) and the United States Environmental Protection Agency (EPA) ( 16 ) (solid lines). Modeled baseline values are averages for the 2020–2025 and 2025–2030 periods as data were produced at 5-year intervals. The shaded area shows the full range across the four to six IAMs for each scenario. Scenario concentration changes are derived from scenario emissions using a simple box model and assumed constant natural emissions of around 200 million tonnes (Mt) yr −1 . Growth rates under 1.5°C-consistent scenarios with policies beginning in 2015 ( 17 ) are also shown for comparison along with their full ranges. Projected rates are compared with observations (circles) from the United States National Oceanic and Atmospheric Administration (NOAA) observation network with 1 standard deviation uncertainties. See Methods (Analysis A) for further details.
Causes of increased methane growth rates and discrepancies with baseline scenarios
Multiple assessments have concluded that the growth in methane concentrations over the 2007–2019 period is largely attributable to increased emissions from fossil fuels and livestock ( 18 – 21 ). However, some studies attribute much of this increase to wetlands (particularly in the tropics)—an attribution potentially supported by isotopic data indicating increased biogenic methane ( 22 – 25 ). In general, longer-term increases in wetland methane emissions (resulting from a human-caused warming climate) are expected to be small over these years as the climate feedback is weak according to models, modern observations, and paleoclimate data ( 19 , 25 – 30 ). Methane emissions associated with thawing permafrost and glacial retreat are also expected to increase as the climate warms, though the magnitude is thought to be small and quite uncertain ( 19 , 31 , 32 ). A small portion of this longer-term increase in the growth rate may be due to growing areas of rice cultivation in Africa ( 33 ). Over the longer 2007–2019 period, there thus remains ambiguity in the cause of observed emission trends given geographical and sectorial methane source diversity.
Investigations into the cause of the large increase in the growth rate in the 2020–2023 period relative to the prior years are just beginning. Some atmospheric-chemistry transport modeling studies have attributed more than half of the increased growth in 2020 relative to 2019 to changes in methane removal owing to a decline in the hydroxyl radical OH driven by COVID-19-related changes in emissions, primarily decreases in nitrogen oxides ( 34 – 36 ). However, other changes that constrain methane removal rates using methane observations attribute just 14–34% of the increased 2020 growth rate to changes in the sink ( 37 , 38 ). The persistence of the very high growth rates in 2021 and 2022 also supports evidence of the role of reductions in OH and methane loss rates driven by COVID-19-related emissions changes. This is consistent with Feng et al. ( 38 ), who found the role of sink changes decreased from ~34% in 2020 to just 10% in 2021. Thus, changes in methane removal appear unlikely to play a dominant role in driving the higher 2020–2023 growth rates.
Sink changes playing a minor role implies that the jump in the growth rate from 7 to 10 ppb yr −1 during the 2015–2019 period to ~12–18 ppb yr −1 during the 2020–2023 period is attributable to increased emissions, which can be examined using “bottom-up” analyses. Emission increases are unlikely to be attributable to the waste or agriculture sectors, which vary minimally from year to year. For example, global cattle numbers grew at an average rate of 1.1% yr −1 over the 2020–2022 period; this was only modestly larger than the 0.9% yr −1 average over the 2015–2019 period ( 39 ). This translates to an increase of <1 Tg yr −1 assuming constant methane emissions per animal, a small fraction of the implied emissions increase ( Figure 1B ) (and in contrast to the longer-term growth in cattle numbers which leads to an increase of ~10 Tg yr −1 over the 2007–2019 period). The more rapid growth of atmospheric methane over the 2020–2023 period therefore appears to be primarily linked to increased emissions from fossil fuels and wetlands, which together may account for the underestimated growth rates in the IAMs ( Figure 2 ).
For fossil fuels, there is evidence that investments in midstream capacity have been inadequate to keep up with the volume of extracted gas as firms ramp up production. For instance, the state-owned oil company in Mexico flared ~63 billion cubic feet of gas from a single field (Ixachi) over the 2020–2022 period, representing more than 30% of the field’s total production and being in violation of Mexican law ( 40 ). Flaring to mitigate methane release is imperfect in the field: aerial measurements over multiple United States oil and gas regions indicate an efficiency of around 91% owing to both incomplete combustion and unlit flares, which, combined with large volumes of flared gas due to midstream capacity shortages, results in large methane emissions ( 41 , 42 ). Studies report inefficient or inactive flares in other regions, such as Turkmenistan ( 43 ).
Additionally, some projections incorporate current emissions from national reporting, whereas studies using atmospheric inversions from satellite data suggest that oil- and gas-extracting countries in central Asia and the Persian Gulf region typically systematically underreport their emissions ( 44 ). This is similar to findings for the United States and Canada ( 45 , 46 ). National reporting also generally omits so-called super-emitters ( 47 – 49 ), which are discussed further below. Large underestimates in initial methane emissions could lead to underestimated emission growth. Discrete events may have also played a role, with the COVID-19 pandemic being linked to increased methane emissions from the energy sector in early 2020 ( 50 ) and the 2022 Russian invasion of Ukraine causing increased efforts to expand supplies of gas and coal ( 51 ). There are thus several reasons fossil fuel emissions might be growing faster than in baseline scenarios.
However, increased methane emissions from wetlands appear likely to have driven a larger portion of the higher 2020–2022 growth rates based on the latitudinal gradients of growth rates and a trend toward lighter (biogenic) isotopes of atmospheric methane ( 52 ). The cause may be in part a persistent La Niña pattern that likely enhanced tropical wetland methane emissions during the 2020–2022 period. The wetland methane increase has been estimated at ~4–12 million tonnes (Mt) yr −1 based on empirical analyses of prior events ( 25 , 53 , 54 ), though another study found a weaker La Niña impact on methane ( 55 ). A recent modeling study shows a rise of ~5 Mt yr −1 in the wetland methane flux for the 2020–2021 period relative to the prior 3 years ( 25 ), predominantly from tropical ecosystems and consistent with satellite studies ( 38 ). Wetlands were also implicated in earlier analyses of the 2020 growth rate increase relative to 2019 ( 35 ), with an especially large increase in emissions from Africa ( 37 ). A rise of ~5 Mt yr −1 would be a relatively modest contribution to the overall jump in emissions estimated at ~30 Mt yr −1 for the 2020–2022 period relative to the prior 5 years ( Figure 1A ). There are, however, substantial uncertainties in terms of tropical wetland methane emissions ( 56 ), and modeled wetland methane emissions may be biased substantially low, especially over Africa ( 57 , 58 ), so the increase shown in the models may be an underestimate. The La Niña is superimposed on anthropogenic warming and changes in climate extremes that could also lead to higher wetland methane fluxes than in previous La Niña events.
A switch from La Niña to El Niño during 2023 appears to have reduced the observed growth rate ( Figure 2 ), supporting a large role for wetland responses to La Niña in the very high 2020–2022 growth rates. However, emissions appear to have remained substantially higher in 2023 relative to pre-2020 values ( Figure 1B ), suggesting longer-term contributions from increasing anthropogenic sources along with a forced trend in natural sources. Recent work also suggests a potentially permanent shift to an altered state of enhanced wetland methane emissions ( 8 ). The next 5–10 years of monitoring will, therefore, be critical in understanding both short- and long-term feedback and drivers of accelerated growth rates. While current estimates suggest increases in fossil fuel emissions, especially wetland methane, likely dominated the growth rate jump after 2019, reconciliation of observed growth rates with emissions inventories remains elusive. Regardless of the relative contribution of the two most probable major sources of the longer-term 2007–2023 increase in growth rates—i.e., wetland feedback from human-driven warming and human-driven emissions—the implications are identical: anthropogenic emissions must decrease more than previously expected to reach a given climate goal.
Imperative 2—to align methane and carbon dioxide mitigation
Methane and co 2 emissions targets.
As methane targets are currently being set in many countries, it is important to understand how these fit within the broader climate change mitigation agenda and the push for “net zero CO 2 ”. Least-cost 1.5°C- and 2°C-consistent scenarios require major and rapid reductions in methane alongside CO 2 ( 4 , 6 , 17 ). For example, AR6 1.5°C scenarios with limited or no overshoot achieve net zero CO 2 emissions around the middle of the century while methane emissions decrease by a mean of 35% (standard deviation: ±10%) in 2030, 46% (±8%) in 2040, and 53% (±8%) in 2050 relative to 2020 levels (Analysis B; Figure 3 ) ( 59 ). Global emissions targets well within these ranges, as in the Global Methane Pledge, are thus aligned with the Paris Climate Agreement. Delaying methane reductions past the timescales in 1.5°C-consistent scenarios risks higher overshoot, peak temperatures, and costs.
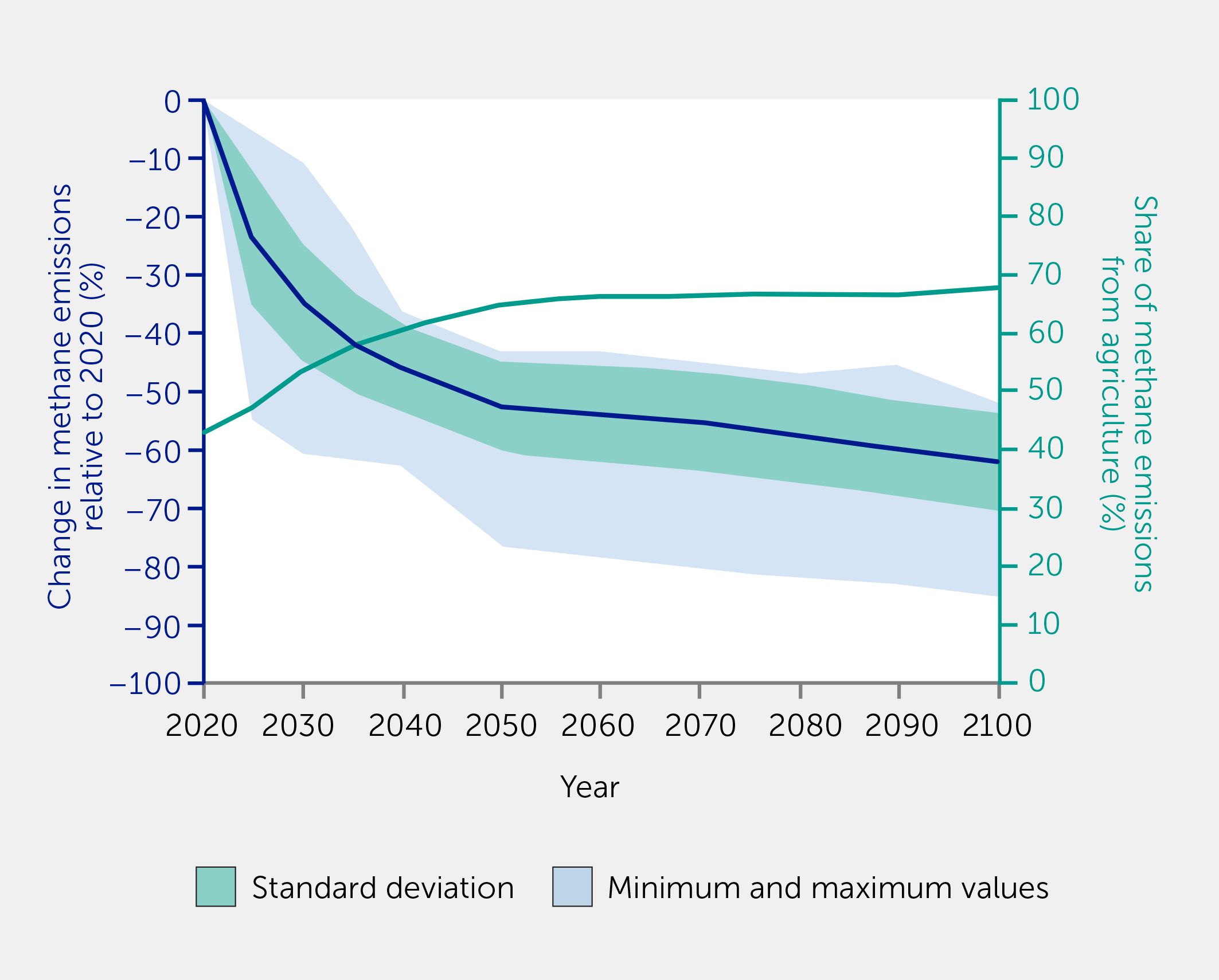
Figure 3 Decrease in total methane emissions and increase in agricultural share of the remainder in 1.5°C-consistent scenarios. Mean decrease in anthropogenic methane emissions relative to 2020 under least-cost 1.5°C consistent scenarios with policies beginning around 2020, including the standard deviation across the 53 scenarios analyzed and the maximum and minimum values across the scenarios. Also shown is the mean share of anthropogenic emissions from the agriculture sector in the same scenarios. All scenarios for which agricultural as well as total emissions were available were included ( 59 ). Note that the median scenario is virtually identical to the mean shown here. See Methods (Analysis B) for further details.
Net zero CO 2 emissions is a relevant concept because options are available currently to drastically reduce CO 2 in almost all emitting sectors, and carbon dioxide removal (CDR) options, including afforestation, exist for the remainder. Removal options are in the early research stages and are not currently available for methane or nitrous oxide (N 2 O). For those gases, we therefore discuss “zero anthropogenic emissions” (i.e., without the “net”).
The vastly different lifetimes of methane and CO 2 lead to markedly different requirements for zero-emission targets. CO 2 , as well as other long-lived greenhouse gases (LLGHGs) such as N 2 O and many fluorinated gases, accumulates in the atmosphere; emissions must thus reach net zero to achieve long-term climate stabilization ( 17 ). In contrast, methane and other short-lived pollutants do not accumulate, and hence long-term climate stabilization requires only constant emissions rather than zero, with weakly decreasing emissions yielding shorter-term stabilization. Consistent with this and owing to the difficulty in reaching zero emissions in some sectors such as agriculture, none of the least-cost 1.5°C-consistent scenarios achieve zero methane ( Figure 3 ).
Discussion of net zero GHG targets could easily be misinterpreted to imply that we can wait to reduce non-CO 2 emissions since those scenarios that do achieve net zero GHGs reach net zero CO 2 first. For long-term climate stabilization, the temperature depends upon the total LLGHGs emitted before reaching net zero along with the continuing short-lived pollutant emissions rate at that time, and there exists a similar relationship for peak temperatures under a peak-and-decline scenario. Article 4.1 of the Paris Climate Agreement calls for “balancing sources and removals of GHGs”, but this applies to all GHGs collectively. Achieving such a balance for methane is neither required under Article 4.1 nor for meeting the temperature goals established in Article 2 of the Agreement. In practice, methane emission projections in 1.5°C-consistent scenarios are substantial through 2100 ( Figure 3 ). Thus, scenarios that achieve net zero GHGs accomplish this not by lowering non-CO 2 emissions to zero but by aggressive deployment of CDR that offsets residual methane and N 2 O. This leads to gradually decreasing warming, a requirement during overshoot scenarios. Reducing warming after reaching net zero CO 2 thus requires CDR, reductions of methane and/or N 2 O, or a combination of these. Such reductions often lead to net zero GHGs by 2100 but not always ( 6 ). This suggests that while net zero GHGs may be a laudable post-net zero CO 2 goal, it might be more useful to focus separately on net LLGHG and methane targets than on net zero GHGs, which combine long- and short-lived pollutants in a metric-dependent way that obscures policy-relevant information ( 60 ) and may not be required or may be insufficient to achieve a given temperature target depending upon prior emissions.
Additionally, residual methane emissions in 1.5°C-consistent scenarios are dominated by the agricultural sector ( Figure 3 ). A net zero GHG target that was interpreted as requiring zero methane could thus lead to conflicts between the pressure to reduce emissions from agriculture and the need to feed the world’s population. Though reducing agricultural emissions of both LLGHGs and methane is necessary and feasible ( 4 , 61 , 62 ), planning for net zero GHGs may lead to unrealistic expectations that could hinder progress in some countries and sectors. We, therefore, recommend that targets be formulated using net LLGHG emissions but total emission levels for short-lived pollutants.
There is an interplay between these two factors, as the higher the level at which emissions of short-lived warming pollutants remain the less total LLGHG emissions are permitted until reaching net zero to achieve a given warming level. This can be quantified using the remaining carbon budget for a particular temperature goal. To have a two-thirds chance of staying below 2°C, the remaining CO 2 budget from 2020 is ~1150 GtCO 2 ( 19 ), assuming roughly 35% reductions in methane by 2050. Every 100 Mt yr −1 of methane not permanently cut would take away about 300 GtCO 2 from the CO 2 budget over the next 50–100 years ( 63 ). This highlights the critical role of methane reductions in facilitating a plausible CO 2 reduction trajectory consistent with the Paris Agreement: the remaining carbon budget would otherwise become too small to make achieving those goals feasible ( 64 , 65 ).
Similarly, the more methane has been reduced upon reaching net zero CO 2 emissions the less CDR would be required. For example, every additional 50 Mt yr −1 of methane permanently reduced would offset the need for ~150 Gt GtCO 2 CDR over the following few decades [and >200 Gt GtCO 2 over the longer term ( 66 )]. Given the many challenges and potential negative impacts of CDR ( 19 , 67 , 68 ), this continues to motivate us to pursue the greatest possible methane reductions.
Measuring progress: methane and CO 2 metrics
In addition to setting sound targets, it is important to use appropriate metrics to measure progress. Evaluations typically use so-called “CO 2 -equivalence” (CO 2 e), which combines all gases using the global warming potential (GWP) at a fixed time horizon, generally 100 years [e.g., ( 66 )]. Using any single timescale to compare short-lived pollutants and LLGHGs provides an incomplete picture [e.g., ( 69 )]. More complete climate information is gained by using multiple timescales ( 70 , 71 ), among other means.
A new metric, GWP*, represents the differing effects of changes in short- and long-lived emissions on future global mean temperatures better than GWP ( 72 ). As such, the GWP* metric captures the 50–100-year relationship between continued methane emissions and the carbon budget. Hence, GWP* can be useful when examining decadal-century scale temperature changes, though multiple metrics better reflect the multiple timescales of potential interest. GWP* is applied to sustained changes in emissions, requiring careful consideration of the fact that every tonne of methane emission that persists decreases the remaining carbon budget.
One could evaluate the contribution of emissions relative to preindustrial levels using GWP*, which would show the large warming impact of present-day methane emissions ( 60 ). However, some countries and companies have used GWP* to suggest that since keeping current methane emissions constant does not add additional future warming, continued constant high levels of methane emissions are therefore not problematic and a reduction of their methane emissions is equivalent to CO 2 removal [e.g., ( 73 – 75 )]. This use of GWP* to justify the continuance of current emission levels essentially ignores emissions responsible for roughly half the warming to date and appears to exempt current high methane emitters from mitigation. This is neither equitable nor consistent with keeping carbon budgets within reach. Many current high emitters are wealthy groups, and the use of GWP* to evaluate changes relative to current levels implies the wealthy consuming or profiting from a large amount of methane-emitting products (such as gas, oil, or cattle-based foods) has no impact, whereas the poor, who currently consume little, would be penalized for consuming more ( 76 ). Policymakers should also consider impacts beyond climate when choosing policies affecting methane ( 4 , 77 – 79 ).
Connections between methane and CO 2 mitigation options
Though the different lifetimes of methane and CO 2 have profound implications for target setting and metrics, the separation between short- and long-lived pollutants is not complete. Much like other short-lived pollutants, methane induces climate changes that affect the carbon cycle—thereby exerting a long-term impact ( 80 , 81 ). This carbon-cycle response to warming adds ~5% to the forcing attributable to methane emissions. Additionally, methane emissions lead to increased surface ozone, which is harmful to many plants and reduces terrestrial carbon uptake. Climate impacts of methane emissions could be increased by up to 10% considering ozone–vegetation interactions ( 12 ).
In addition to these Earth system interactions, mitigation options also link methane and CO 2 . Decarbonization policies phasing out fossil fuels would clearly reduce fossil sector methane emissions. However, those reductions would produce only about one-third of the methane reductions in 1.5°C scenarios by 2030 ( 4 , 82 ). The use of non-fossil methane sources for energy production also modestly reduces CO 2 emissions by displacing demand for fossil fuels, adding ~10% to the long-term and ~3% to the near-term climate effect of methane capture. Other estimates suggest that using non-fossil methane for power generation could increase the monetized environmental benefits of methane capture even further—by 14% and 25% for discount rates of 4% and 10%, respectively. These larger values reflect the inclusion of both climate and air pollution damages and stem primarily from reduced air pollutants associated with coal burning ( 78 ).
Another intersection between decarbonization and methane could occur in a hydrogen economy. Fugitive methane emission rates above ~2% would cancel the near-term climate benefits of “blue hydrogen” with carbon capture and sequestration (CCS) compared to burning natural gas ( 83 ). Furthermore, hydrogen leakage would extend methane’s lifetime by lowering the atmospheric oxidative capacity [e.g., ( 84 , 85 )].
Land use also links mitigation options for methane and CO 2 . There are large land area requirements for either bioenergy with CCS (BECCS) or afforestation, two sources of CDR that most low-warming scenarios require to compensate for slow decarbonization and/or continued emissions from the sectors most difficult to decarbonize ( 17 ). Given the demands on arable land to feed a growing population and the urgent need to restore and conserve biodiversity, a plausible source of additional land is reduced numbers of pasture-raised livestock, which could also reduce methane emissions.
To probe this connection, we examined 145 least-cost 1.5°C scenarios for which trends in pasture area and BECCS deployment were available (Analysis C) ( 86 ). The deployment of BECCS closely mirrors a decline in pasture area in these scenarios ( Figure 4A ), a relationship noted but not quantified in AR6 ( 59 ). Examining the multi-model mean decadal changes from the 2040s onwards, when deployment of BECCS is large enough to show clear trends, we find highly correlated changes, with every 10 exajoule (EJ) of BECCS associated with ~38 million ha pasture area decrease ( Figure 4B ) and ~0.5 Gt yr −1 CO 2 removal. Adding in the 2030s increases the slope to 42 million ha per 10 EJ, whereas examining each individual scenario’s changes, rather than the multi-model mean, shows the slope is 28 million ha per 10 EJ. These comparisons give a sense of the robustness associated with this relationship.
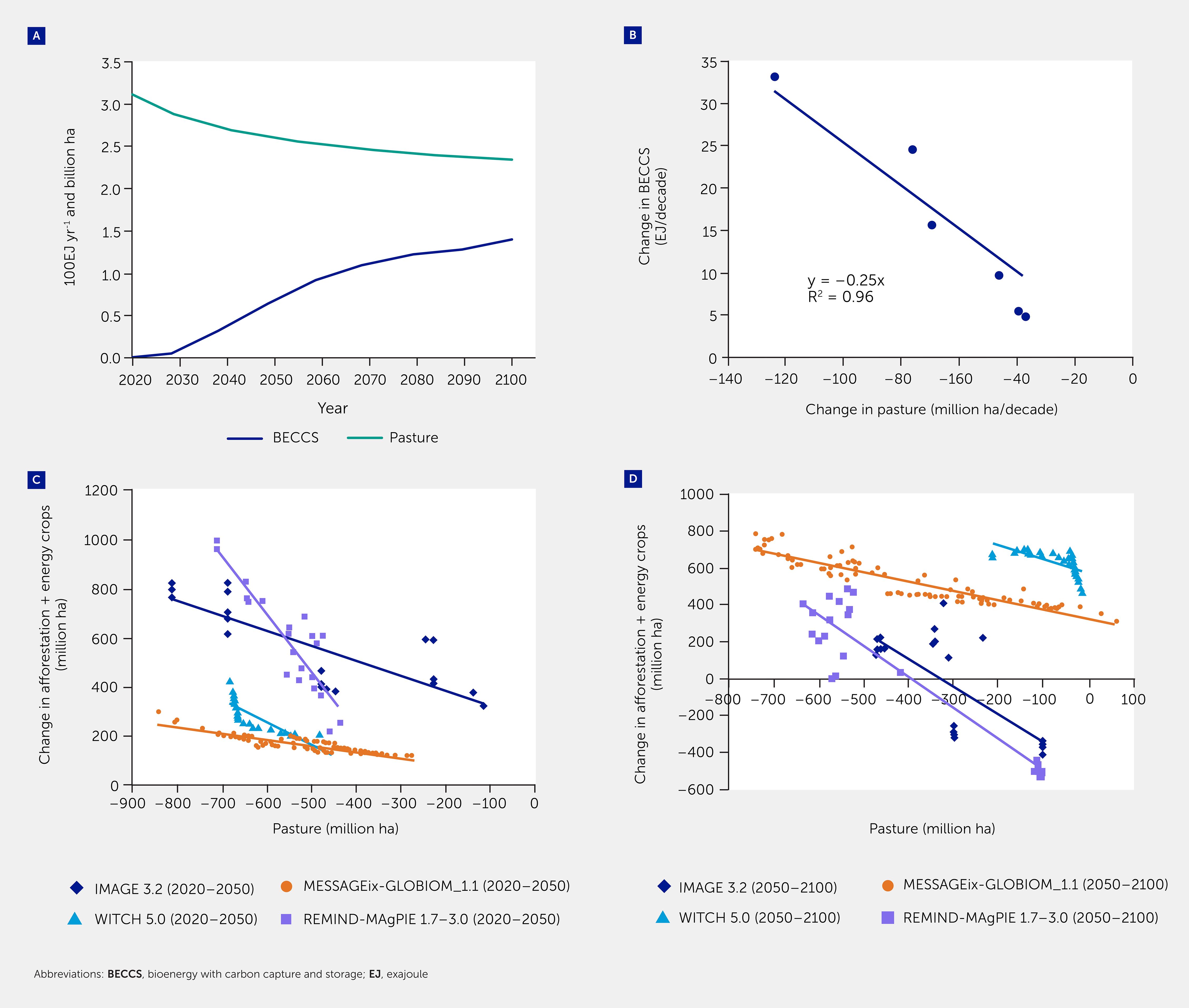
Figure 4 Trade-offs between land use for pasture and for carbon uptake. (A) Multi-model mean trends in bioenergy with carbon capture and storage (BECCS) deployment and in pasture area in the 145 available least-cost 1.5°C scenarios. (B) Correlation between decadal changes in the multi-model means of these two quantities from the 2040s to 2090s. Data are from seven integrated assessment models (IAMs) from the 2022 AR6 scenario database ( 86 ). Also shown are land use changes from simulations covering 22–106 <2°C scenarios per model in individual IAMs for 2020–2050 (C) and 2050–2100 (D) , including linear trend estimates across the scenarios. See Methods (Analysis C) for further details.
For reforestation and afforestation, meeting goals in national climate pledges is projected to require almost 1.2 billion ha of land ( 87 ). For context, the current crop area is about 1.2 billion ha (including animal fodder), so changes in land used for crops for humans would be too small to provide the land needed while maintaining food security. While some land needs might be met via restoration of degraded lands, more than half was estimated to require conversion of pasture or land currently used for animal fodder.
To evaluate the relationship between afforestation plus biofuel land use and pasture, we examined a larger AR6 set of scenarios that keep warming below 2°C, finding 266 scenarios (Analysis C). Averaged across the models, pasture area decreases by 1.1 ha per 1 ha land used for carbon uptake from 2020–2050 and by 0.6 ha from 2050–2100. Assuming carbon uptake per ha biofuel crops is similar to afforestation, this corresponds to ~94 million and 54 million ha of pasture required per GtCO 2 removal, with a range of 28–251 million ha across the models. This range encompasses the results based on BECCS alone in the 1.5°C scenarios. Together, these analyses show robust evidence of a tradeoff between land used for CDR and pasture with a value that is highly model-dependent. In the four models including afforestation, changes in land deployed for carbon uptake are highly correlated with pasture decreases across the scenarios, with R 2 >0.6 and 0.4 for 2020–2050 and 2050–2100, respectively ( Figures 4C, D ). Within the IAMs, MESSAGE and REMIND show fairly linear relationships whereas the land use tradeoff is more dependent on the scenario in WITCH and IMAGE ( Figures 4C, D ). Land for CDR is used primarily for BECCS in MESSAGE and WITCH, primarily for afforestation in IMAGE, and comparably for those options in REMIND, highlighting that the tradeoff with pasture holds for all uptake options deployed in the models. Inter-model differences presumably stem from varying assumptions about the availability of non-agricultural land for afforestation, changes in non-energy crop area, and the intensity of carbon uptake via afforestation or energy crops.
The results show that shifting livestock practices, especially healthier dietary choices that in many places lead to reduced consumption of cattle-based foods and hence decreased livestock numbers, not only affect methane emissions but are also tightly coupled with CDR strategies ( 88 ). Both current pledges for biological carbon removal and BECCS deployment at the scales envisioned in many scenarios likely require large reductions in pasture area, and dietary changes could free up pasture without risking food security. We note that both biological carbon removal and BECCS come with substantial challenges and side effects that affect the likelihood that they will ever be societally acceptable at scale ( 19 , 87 ).
In summary, reductions in methane emissions are not just complementary to CO 2 reductions but can directly contribute to reduced atmospheric CO 2 via carbon cycle interactions and fossil fuel displacement. They can also potentially play an important role in facilitating the deployment of, as well as reducing the need for, CDR; this could reduce additional feedback, including increased volatile biogenic compound emissions following afforestation that might increase methane’s lifetime ( 89 ).
Impacts of methane and carbon dioxide mitigation
As noted, methane emissions are estimated to account for 0.5°C of the total observed warming of 1.07°C through the 2010–2019 period ( 1 ). As the climate is affected by both warming and cooling pollutants, the attribution of the fraction of observed warming to a specific component depends on which drivers are included in the comparison. Compared with the total observed warming, methane emissions are responsible for ~47% of that value; in comparison with the warming attributable to all well-mixed GHGs, methane emissions are responsible for ~34%; and in comparison with the temperature increase due to all warming agents, methane emissions contribute ~28%. As the overlap between methane sources and other climate drivers is relatively limited, methane could potentially be reduced with only modest effects on other emissions. Comparison with observed net warming may therefore be most useful, but each of these comparisons is useful for specific purposes. To prevent public confusion, presentations that imply methane’s contribution is being evaluated against observed warming when it is not and that do not state if they are referring to emissions or concentrations, such as the common statement that methane is responsible for around 30% of global warming since pre-industrial times [e.g., ( 90 , 91 )], should be avoided. Note also that the share of warming attributable to a given driver varies depending upon the baseline period (1850–1900 in AR6).
Emission reduction policies that target methane and CO 2 have complementary and additive benefits for the climate. We analyzed the response of global mean annual average surface air temperatures to emissions under various scenarios to isolate the effects of decarbonization and targeted methane emission controls (Analysis D). Contemporaneous reductions in cooling aerosols associated with decarbonization lead to modest net warming over the first few decades [e.g., ( 13 , 92 – 95 )]. Given the smaller role of other non-CO 2 climate pollutants, methane emission cuts therefore provide the strongest leverage for near-term warming reduction ( Figure 5 ) ( 13 , 95 ). Achievement of methane reductions consistent with the average in 1.5°C scenarios could reduce warming by ~0.3°C by 2050 in comparison with baseline increases ( 4 ). A hypothetical complete elimination of anthropogenic methane emissions could avert up to 1°C of warming by 2050 relative to the high emissions Shared Socioeconomic Pathway [SSP; ( 96 )] SSP3–7.0 scenario ( 97 ). This large near-term impact partly reflects methane’s short lifetime; >90% of increased atmospheric methane would be removed within 30 years of an abrupt cessation of anthropogenic emissions compared with only ~25% of increased CO 2 following CO 2 emission cessation ( 98 ). Encouragingly, were humanity to abruptly cease emissions, the present combined anthropogenic CO 2 and methane concentration increases versus preindustrial [weighted by their warming contributions, including the ozone response to methane ( 12 )] levels would be halved within 30 years. Hence the near-term “Zero Emissions Commitment” of warming already “in the pipeline” ( 19 , 99 ) is much smaller considering both methane and CO 2 rather than CO 2 alone.
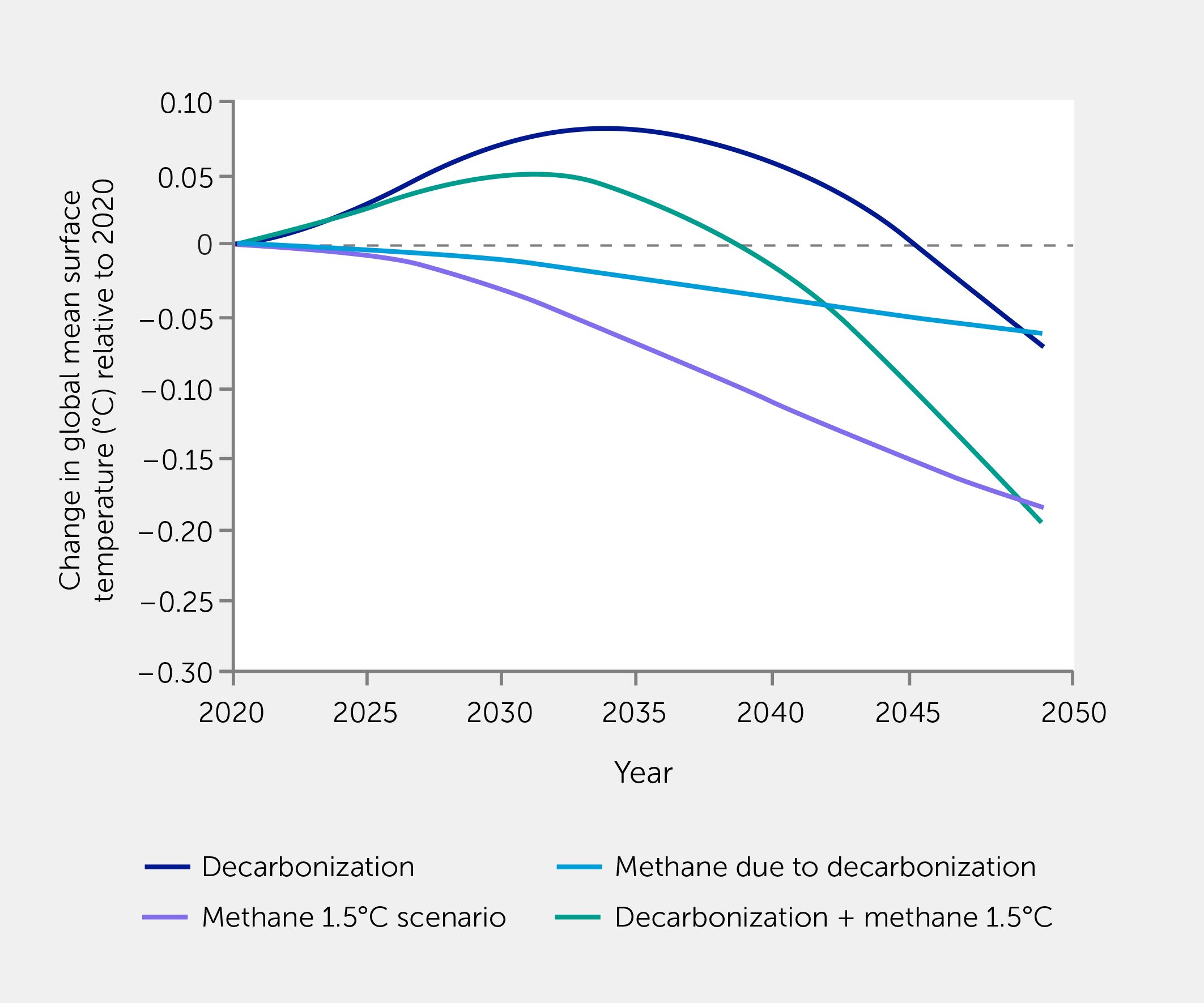
Figure 5 Climate impacts of decarbonization and methane reductions. The climate response (measured by change in global mean surface temperature relative to 2020 values) to reductions of all pollutants (including methane) under a decarbonization scenario; methane alone under a decarbonization scenario that substantially reduces energy sector emissions and under a 1.5°C scenario; and decarbonization and methane reductions consistent with 1.5°C—all relative to constant 2020 emissions. Values are averages across Shared Socioeconomic Pathways (SSPs) 1, 2, and 5 (1.5°C was infeasible under SSP3 in four of four models and under SSP4 in two of three models). See Methods (Analysis D) for further details.
Policies leading to rapid and deep cuts in both CO 2 and methane provide the strongest benefits across the century ( Figures 5 ; 6A ). To further characterize the relative contributions, we analyzed temperature responses, and their effects on premature mortality, applied to various mitigation options under the “middle-of-the-road” SSP2 (Analysis E). Importantly, future CO 2 emissions exert the strongest leverage on long-term climate change, and successfully targeted methane reduction without simultaneous CO 2 reductions over the next 10–30 years would therefore merely delay long-term warming ( Figure 6A ). Conversely, successful reduction of CO 2 (and co-emissions) without simultaneous additional targeted methane reduction over this period would weakly affect long-term temperatures if methane reductions were achieved later ( Figure 6A ) but would lead to higher warming and substantially increased risk of overshooting warming thresholds over the next few decades. In addition to the impacts on warming, a 20-year delay in methane reductions from 2020 to 2040 would also lead to 4.2 (1.3–6.8; 95% confidence) million additional premature deaths due to ozone exposure by 2050 that could have been avoided with rapid methane reductions based on our standard epidemiological estimates ( Figure 6B ). That value becomes ~8.8 (5.5–11.1) million additional deaths using alternative cardiovascular and additional child-mortality relationships (Analysis E).
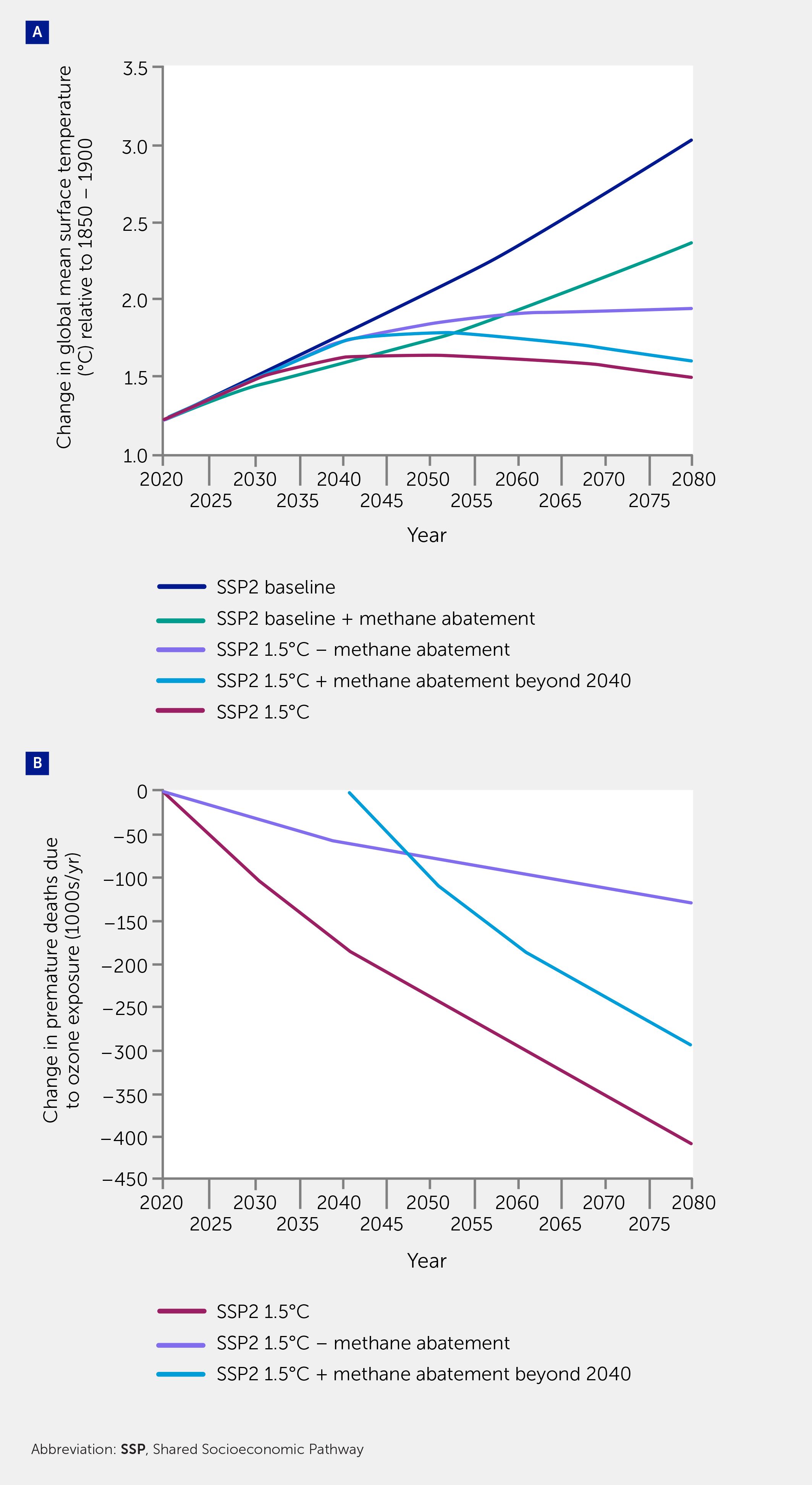
Figure 6 Temperature and health impacts of methane abatement under various scenarios. (A) Climate response (measured by change in global mean surface temperature relative to 1850–1900 values) to all pollutants under the baseline Shared Socioeconomic Pathway (SSP) 2 scenario; the SSP2 baseline plus methane abatement consistent with a 1.5°C scenario; the SSP2 1.5°C scenario (SSP2–1.9); the SSP2 1.5°C scenario without any additional methane abatement beyond that occurring due to the phase-out of fossil fuels; and the SSP2 1.5°C scenario with additional methane abatement beyond that occurring due to the phase-out of fossil fuels beginning in 2040 rather than 2020. (B) Avoided premature deaths resulting from methane reductions relative to those under the SSP2 baseline (note that SSP2 baseline plus methane abatement consistent with a 1.5°C scenario is identical to the SSP2 1.5°C scenario for this impact and so is not shown). See Methods (Analysis E) for further details.
In addition to reducing early deaths, cutting methane emissions will reduce near-term warming impacts on labor, which grow non-linearly with warming ( 100 ). We used our climate Analysis E as the basis to estimate corresponding labor effects of changing heat exposure (Analysis F). Assuming outdoor workers are in the shade, achieving 1.5°C-consistent methane abatement under SSP2 avoids roughly US$250 billion in worldwide potential heavy outdoor labor losses by 2050 (range US$190–US$390 over impact functions; values in 2017 US$ purchasing power parity). However, for outdoor workers in the sun, benefits would be roughly US$315 billion (range US$211–US$475). These values, for heavy outdoor labor only, are not comparable to impacts covering medium and light labor (for which the evidence base is weaker).
Imperative 3—to optimize methane abatement options and policies
Global context.
Despite substantial uncertainties in emissions from specific subsectors, global-scale anthropogenic methane emissions are reasonably well-constrained. Agriculture and fossil fuel emissions have comparable magnitudes (each ~130–150 Mt yr −1 ) roughly twice that of the waste sector (~70–75 Mt yr −1 ) ( 4 , 101 ). Abatement technologies are available in each sector ( 102 ) and, with modest projected improvements over time, could provide reductions of 29–62 Mt yr −1 in the oil and gas subsectors together, 12–25 Mt yr −1 in the coal subsector, 29–36 Mt yr −1 in the waste sector, and 6–9 Mt yr −1 from rice cultivation in 2030 ( 4 , 90 ). Estimated abatement for livestock ranges from 4–42 Mt yr −1 , depending upon factors such as the assumed potential to adopt higher productivity breeds and/or reduce total animal numbers. Technical abatement could be enhanced with nascent technologies such as methane inhibitors for ruminants, cultured and alternative proteins, and, in the waste sector, biocovers, black soldier flies, and waste-to-plastic substitute systems.
Many technological abatement options capture concentrated flows of methane, allowing it to be used as natural gas, generating revenue that lowers net costs. Defining low-cost as <US$600 per tonne of methane (in 2018 US$), low-cost abatement potentials represent 60–98% of the total for oil/gas, 55–98% for coal, and ~30–60% for waste ( 4 , 89 ). Technical options with net negative costs could reduce total emissions by ~40 Mt yr −1 , with the greatest potential being in the oil/gas and waste sectors ( 4 ).
Systemic and behavioral choices, such as fuel switching and demand management, also affect methane emissions and are particularly important in the food sector. Cattle account for about 70% of livestock emissions, with ~25% from regions with high reliance on intensive systems (primarily Europe and North America) most suitable for technical solutions ( 15 ). In other areas, extensive grazing systems are common, limiting technical solutions ( 61 ). For sizeable reductions in livestock emissions, cuts in animal stocks will therefore be necessary. Shifts to more plant-based diets could bring health benefits in regions with high intake of animal protein ( 103 , 104 ), and, as discussed above, this is important for providing areas for CDR deployment. Such shifts could reduce methane emissions by ~15–30 Mt yr −1 over the coming ~10–25 years ( 4 ). In regions with low protein intake but large cattle herds, productivity should be increased in conjunction with enhancement of the economic resilience of pastoralist communities ( 105 ). The latter requires improved access to affordable healthcare, education, and credit markets to enable management of financial risks without reliance on large livestock herds.
Achieving ~40–50% reductions in food loss and waste could reduce ~20 Mt yr −1 of methane emissions ( 4 ). Systemic and behavioral changes, such as dietary shifts and reduced food loss/waste (DFLW), are often difficult to implement but are benefiting from growing attention. Together, these could substantially augment the 120 Mt yr −1 achievable through targeted technical controls ( 13 , 62 , 106 ). Similarly, the IPCC assessment indicates a mitigation potential from DFLW for all GHGs of about 7 (3–15; full range) GtCO 2 e yr −1 by 2050, of which 1.9 GtCO 2 e yr −1 comes from direct emissions [largely non-CO 2 ( 6 )]. The latter would correspond to ~70 Mt yr −1 methane were it all methane, highlighting the large mitigation potential from DFLW both via methane and via associated land use changes.
National mitigation options: abatement potential and cost-effectiveness by country
The GMP has raised ambition worldwide but achieving its goal requires optimizing efforts, as political and financial capital is limited and time is short. We have therefore undertaken national-level analyses (Analyses G–H) of technical mitigation options for countries seeking to implement the Pledge or non-signatories that may want to reduce their emissions (e.g., China published a National Methane Emissions Control Action Plan in 2023). These analyses may also help optimize international financing. They are based on data from the United States Environmental Protection Agency (EPA) ( 16 ) and the International Energy Agency (IEA) ( 90 ).
Mitigation options with greatest abatement potential by country
Analyses of technological mitigation potential highlight the need to address all subsectors given that each is the largest in at least some countries (Analysis G; Figure 7 ). In some fossil-fuel-producing countries, the greatest opportunities for methane mitigation are in gas and oil whereas coal predominates in other countries. Despite substantial fossil fuel industries, several countries in the Middle East, Southern Africa, and South America are estimated to have their largest mitigation potential in landfills. With few fossil fuels produced outside Eastern Europe and limited technical mitigation potential for livestock, the largest potential for mitigation in Europe is also often in landfills. There are notable exceptions, however. In France, Germany, and the Nordic countries, for example, policies have greatly mitigated waste sector emission, and the livestock subsector now has the largest remaining mitigation potential. This illustrates how national-level data reveal substantial variations even within relatively small geographic regions.
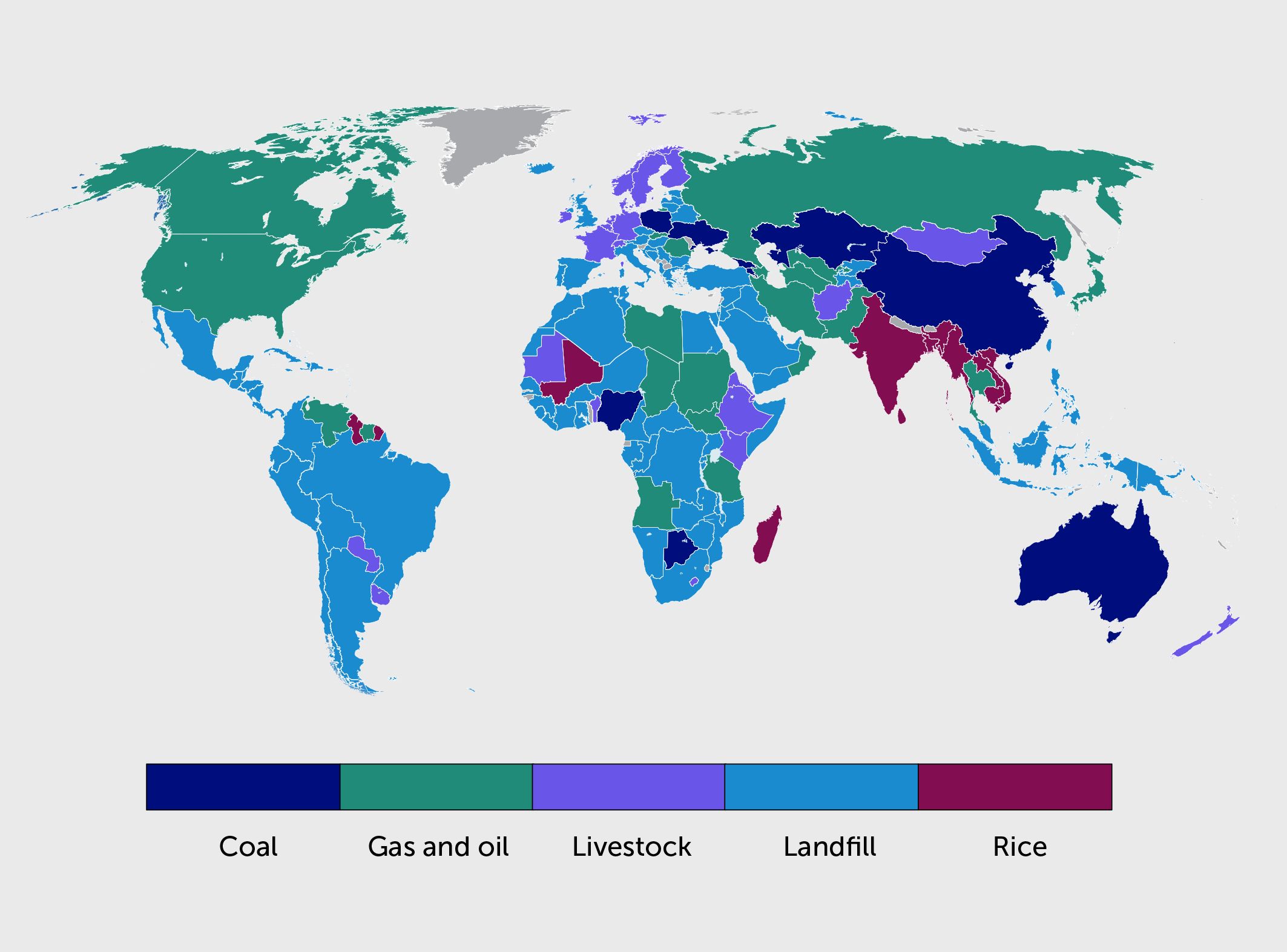
Figure 7 The subsector with the largest technical mitigation potential in every country. The map shows the subsector with the greatest mitigation potential regardless of the cost in each country based on United States Environmental Protection Agency (EPA) data ( 16 ). See Methods (Analysis G) for further details.
This analysis is based on bottom-up emission estimates relying on activity data combined with emission factors. This is the most detailed emission information available by subsector for all countries. However, this approach has uncertainties and limitations. Recent developments in satellite remote sensing have shown the existence of so-called “super-emitters” ( 48 , 49 , 107 ). These are facilities emitting enormous amounts of methane, often related to abnormal operating conditions such as gas well blowouts ( 108 ) or non-burning flares. Hundreds of super-emitters are detectable globally, with even more at local scales [e.g., ( 47 )]. Many super-emitters can be considered “low-hanging fruit” since they are especially cost-effective to mitigate and have high reduction potential per individual source, making them a high-priority category to address. However, they are often not well represented in bottom-up inventories and do not necessarily follow the prioritization per country suggested by the bottom-up analysis ( Figure 7 ). For example, satellite-based studies show emissions from super-emitters from the oil and gas industry in Algeria of ~100 kt CH 4 yr −1 ( 48 , 49 ), a substantial fraction of the estimated mitigation potential not including super-emitters ( Figure 8A ). Super-emitters have also been reported in the coal subsector in Australia, China, and the United States ( 108 – 110 ). Urban areas are also important emission sources that can be difficult to capture in inventories with >13 urban methane hotspots detected in India ( 49 ) and evidence of worldwide urban wastewater emissions hotspots ( 111 ). Based on high-resolution satellite observations, individual landfills in New Delhi and Mumbai were estimated to emit 23 (14–33) and 86 (53–228) kt CH 4 yr −1 ( 112 ), a large fraction of total emissions from their respective urban areas.
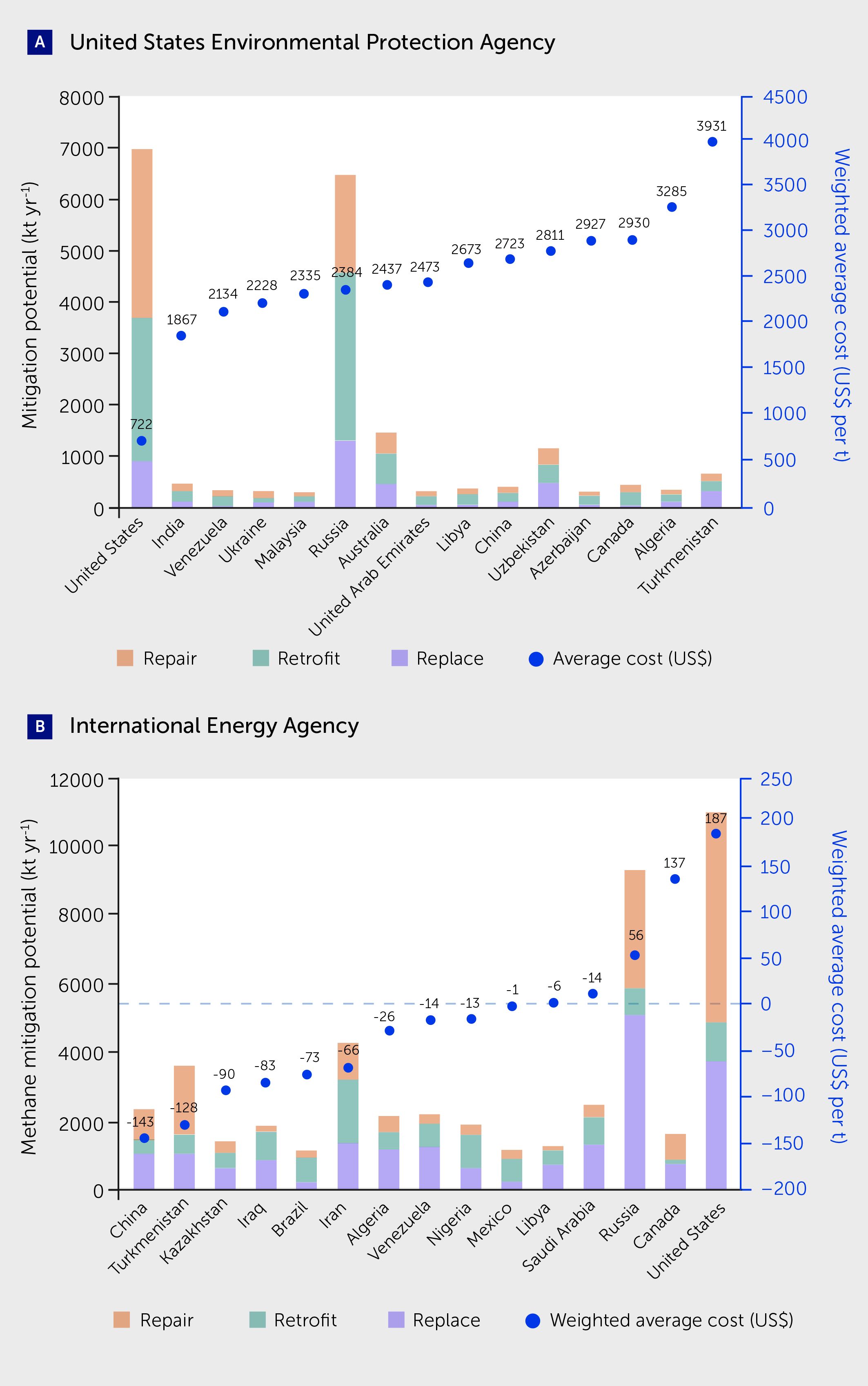
Figure 8 Favorable countries for mitigation of methane from the oil and gas subsector. Estimated methane mitigation potential and costs within the oil and gas subsector for the 15 countries with the greatest mitigation potential in this subsector regardless of costs. Analyses based on data from (A) the United States Environmental Protection Agency (EPA) for 2030 ( 16 ) and (B) the International Energy Agency (IEA) for 2022 ( 90 ). See Methods (Analysis H) for further details.
Mitigation potential and cost-effectiveness by sector and country
To explore cost-effectiveness, we focus on the 50 countries with the largest subsector mitigation potential in the next decade and then rank those by abatement costs (Analysis H). This excludes the agricultural sector due to the limited potential for technical solutions to achieve sizable reductions in the short term. Although this analysis highlights the nations with the largest mitigation potentials at the least average cost, costs vary within each subsector. We therefore created an online tool to explore such details ( https://github.com/psadavarte/Methane_mitigation_webtool ). Mitigation options are grouped into functionally similar categories to facilitate readability and allow comparison across estimates ( Table 1 ).
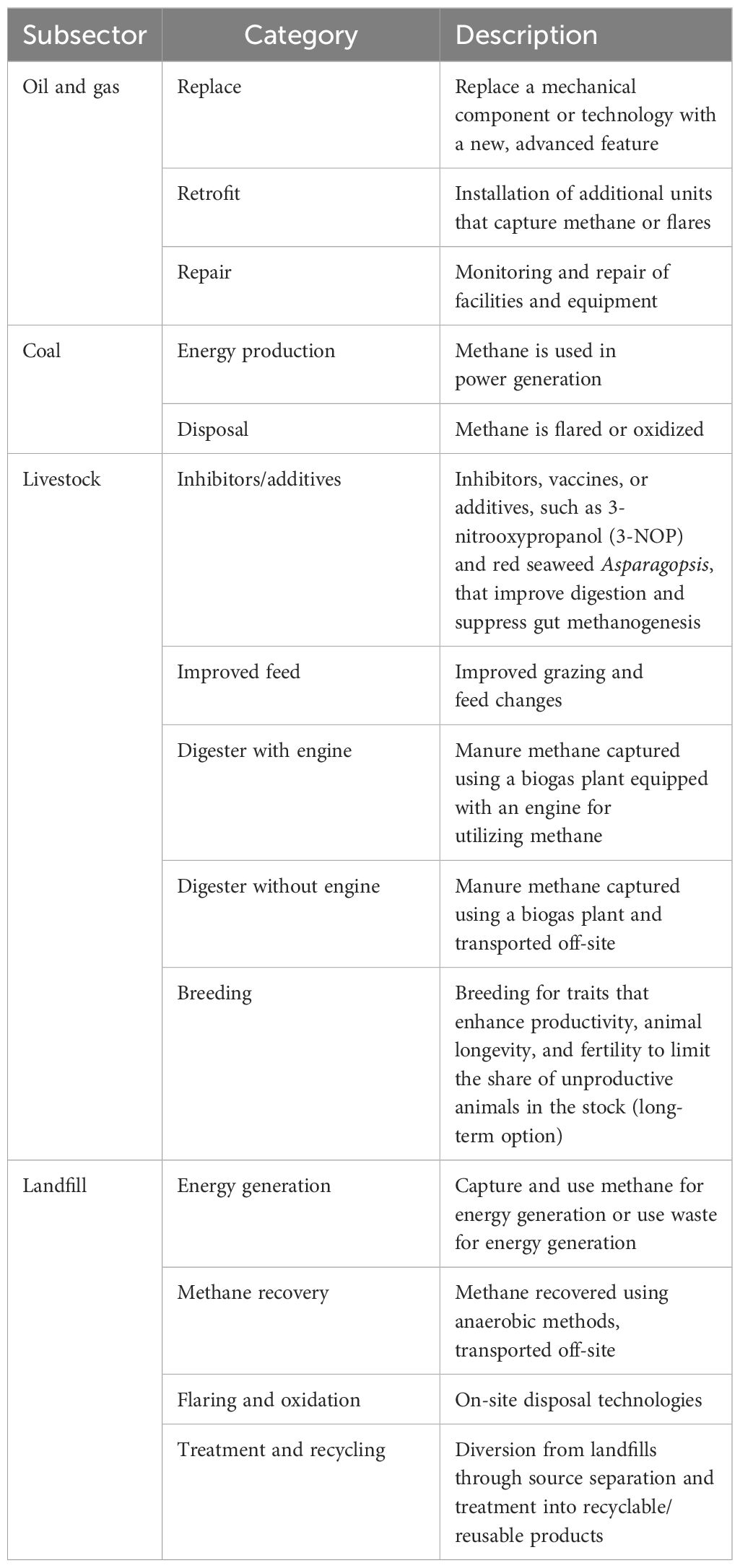
Table 1 Technical mitigation options included in each category.
For landfills, the 15 most cost-effective large reductions total >6 Mt yr −1 , and all have net negative costs ( Figure 9 ). These savings result from revenues provided by methane recovery for use offsite or energy generation. Within these two categories, net mitigation costs range from −US$800 to −US$4400 per tonne. The mitigation potential is always the largest in the energy generation category, hence savings outweigh expenses from flaring and oxidation (~US$120–US$330 per tonne in these countries) and waste treatment and recycling (US$400–US$1700 per tonne). Mitigation potentials are large for some countries with very large populations, such as India, Brazil, and Mexico, but also for several countries with smaller populations including Azerbaijan, Poland, Peru, and the United Arab Emirates. Note that the most cost-effective options do not always have the greatest mitigation potential (e.g., energy generation versus organics diversion).
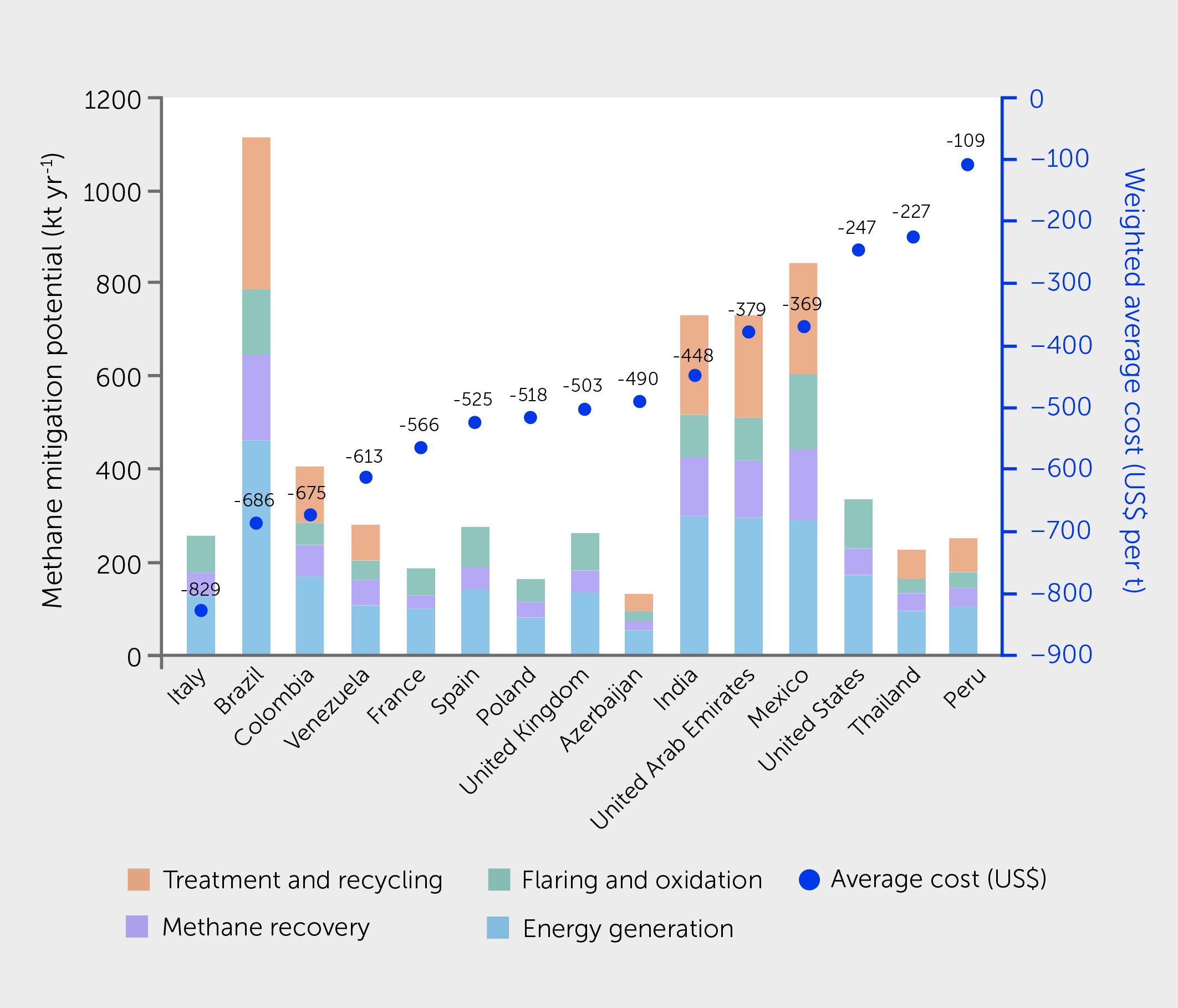
Figure 9 Favorable countries for mitigation of methane from landfills. Estimated 2030 methane mitigation potential and costs within the landfill subsector for the 15 countries with the least expensive average costs that are also among the top 50 countries for mitigation potential in this sector. Analysis based on data from the United States Environmental Protection Agency (EPA) ( 16 ). See Methods (Analysis H) for further details.
Estimating landfill mitigation potentials requires assumptions about waste diversion potentials that are difficult to constrain. For example, analyses by the International Institute for Applied Systems Analysis (IIASA) ( 15 ) for India and China find mitigation potentials ~3.5 times larger than EPA values ( Table 2 ). In contrast, the IIASA mitigation potential for the former Soviet Union countries is smaller. Differences are related to IIASA using both population and economic growth as drivers for waste generation (EPA uses population growth only) and IIASA finding a larger mitigation potential from diversion of organic waste through recycling and energy recovery than in the EPA analysis. National-level analyses have substantially larger ranges in estimated mitigation potentials than the global totals—which are similar to the EPA and IIASA analyses. Cost differences between these analyses are even more striking ( Table 2 ) and reflect differences in the assumed value of recycled products recovered from municipal waste and discount rates (5% for EPA, 4% for IIASA). A small number of very expensive controls in the EPA analysis also have an outsized impact. For example, screening out options costing >US$600 tCH 4 −1 reduces the cost averaged over the remaining measures to −US$2700 tCH 4 −1 for India, closer to the IIASA results.
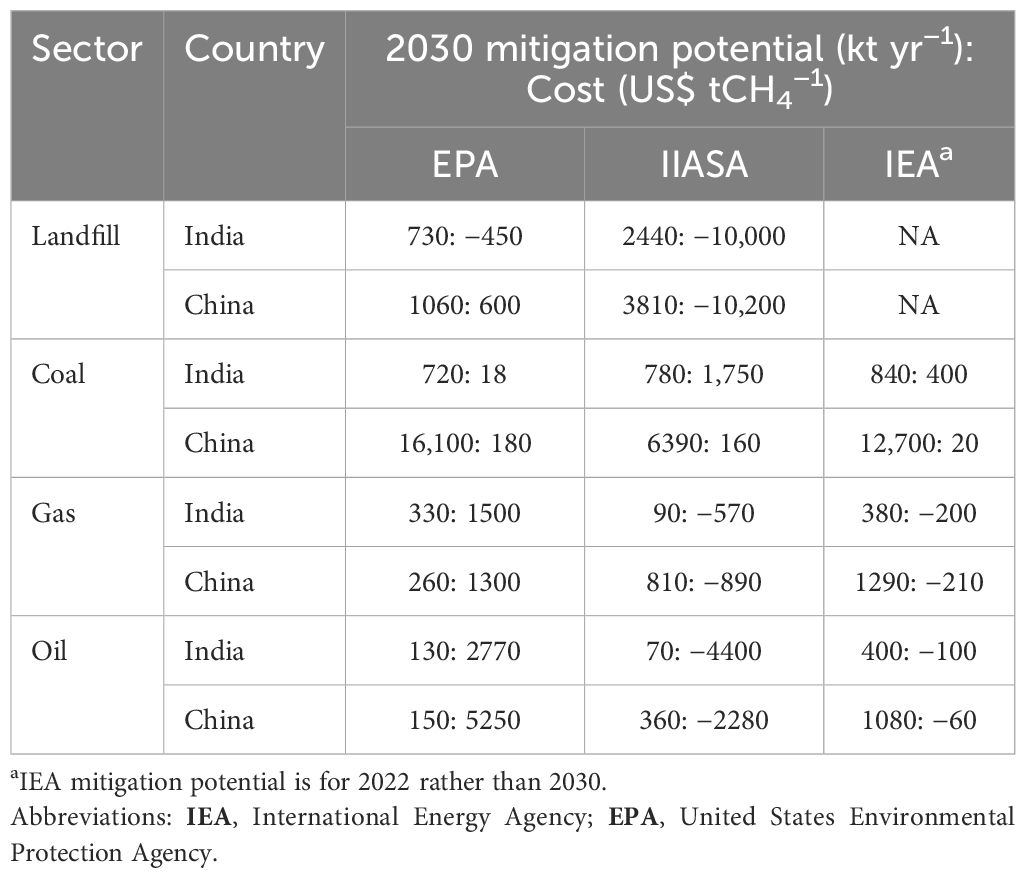
Table 2 Comparison of national data for India and China across available analyses.
For coal, nearly all the most cost-effective large national reductions have positive average costs, though they are low at <US$600 tCH 4 −1 for the top 15 nations ( Figure 10 ; Table 2 ). Mitigation potential in coal within China provides over half the global total for the subsector in all analyses, but the EPA mitigation potential is more than double the IIASA’s, with the IEA being in between ( Table 2 ). The EPA analysis has larger baseline methane emissions from coal in China: 26 Mt yr −1 in 2020 versus 20 and 21 Mt yr −1 in the IIASA and IEA analyses, respectively (2030 values are similar). The lower values are closer to recent satellite inversion estimates of ~16–18 Mt yr −1 ( 113 ). IIASA also makes more conservative assumptions than EPA regarding the fraction of ventilation air methane (VAM) shafts with CH 4 concentration levels high enough (>0.3%) to install self-sustained VAM oxidizers. Cost estimates for China are similar between EPA and IIASA, with the IEA’s being lower. In contrast, the three estimates for coal mitigation potential in India are very similar, but cost estimates differ greatly ( Table 2 ). IIASA’s high costs for India reflect the low VAM concentration there (<0.1%), severely limiting the applicability of oxidizers. Furthermore, abatement potentials in India are similar in magnitude but represent very different percentages of the baseline emissions, with the EPA estimate being roughly one-third that of the other analyses.
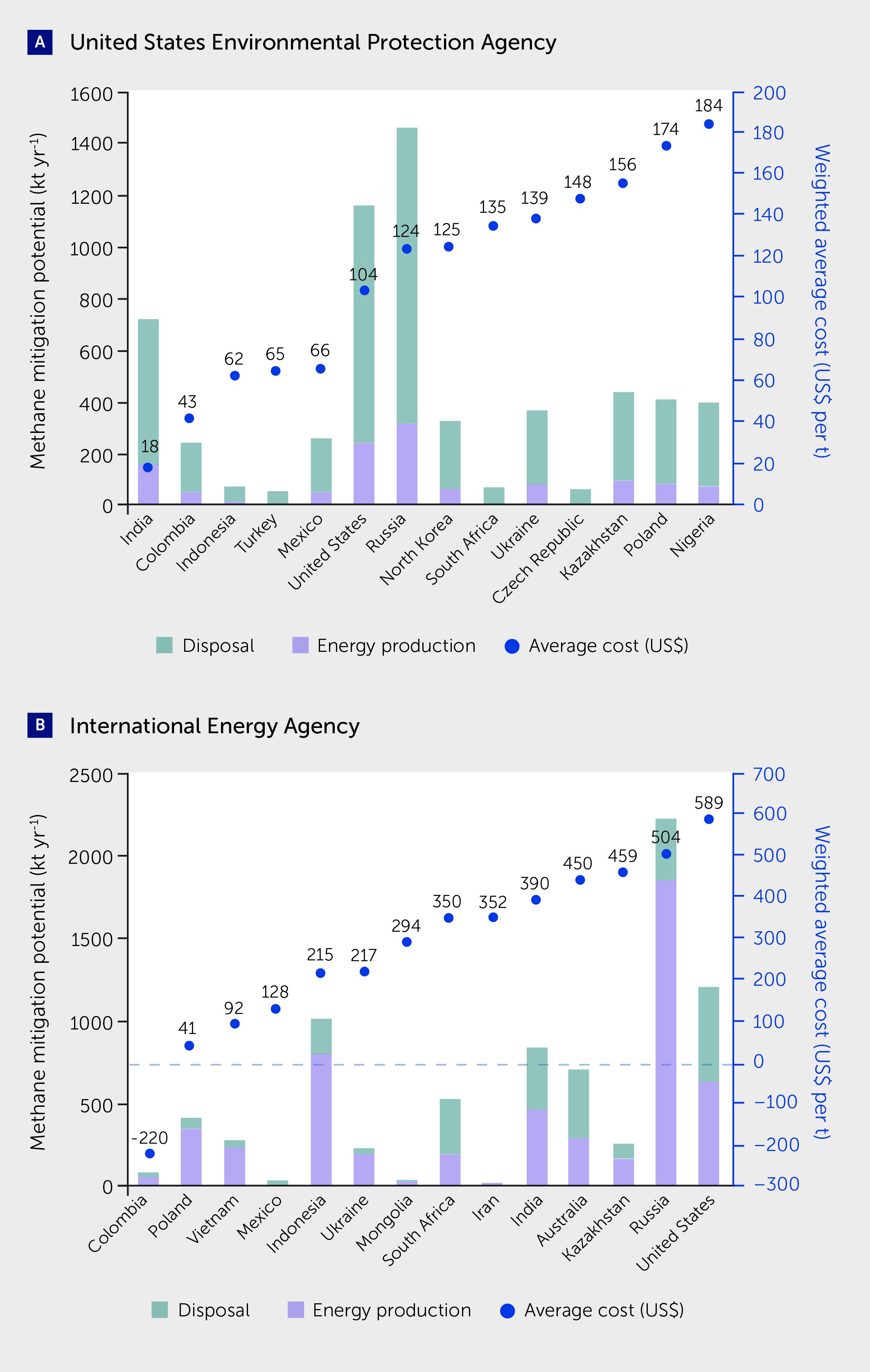
Figure 10 Favorable countries for mitigation of methane from the coal subsector. Estimated methane mitigation potential and costs within the coal subsector for the 14 countries with the least expensive average costs that are also among the top 50 countries for mitigation potential in this subsector. Analysis based on data from (A) the United States Environmental Protection Agency (EPA) for 2030 ( 16 ) and (B) the International Energy Agency (IEA) for 2022 ( 90 ). Note that China is also among the top 15 countries in both analyses but has a mitigation potential of >12,000 kt yr −1 ( Table 2 ), far beyond the scales shown here. See Methods (Analysis H) for further details.
Generally, the EPA estimates lower costs than the IEA, but many countries have similar abatement potentials, including Russia, India, and the United States ( Figure 10 ). In other cases, they estimate extremely different mitigation potentials, for example, in Indonesia and Australia. Differences result from multiple factors, including limited data on base costs and emissions levels, reference years, and technical and economic assumptions. For example, the contrast for Indonesia reflects differences in estimated baseline levels of emission, with the EPA indicating a much lower volume. This may be related to differences in the reference year, with the IEA estimate being more recent and reflecting higher coal activity in Indonesia. Additionally, the EPA uses lower IPCC default emission factors and country-level reporting data to estimate coal mine methane emissions, whereas the IEA considers coal rank, mine depth, satellite measurements, and regulatory frameworks. Finally, the energy production category typically has lower costs than the subsector average, and often net negative costs, whereas the disposal category does not generate revenue and so has higher costs. The latter is typically the largest component in the EPA analysis whereas the former tends to be the largest in the IEA analysis ( Figure 10 ).
Oil and gas
Oil and gas data are available for most countries from the EPA and the IEA. We focus on the 15 countries with the largest potentials regardless of cost because these are similar sets of countries, whereas the most cost-effective within the top 50 differ greatly in these analyses. The comparison shows that 8 countries are among the top 15 by mitigation potential in both analyses, yet these differ markedly in mitigation potentials and especially in mitigation costs ( Figure 8 ). For example, both analyses show the largest abatement potentials in the United States, followed by Russia. However, the potentials estimated by IEA are 40–50% larger than the EPA estimates, while the costs are four-fold lower for the United States and 40-fold lower for Russia. Mitigation potentials diverge even more in other countries. For instance, for Turkmenistan, the IEA finds the potential to mitigate 77% of 4700 kt yr −1 whereas the EPA finds a mitigation potential that is 37% of 1800 kt yr −1 . The IEA analysis, incorporating satellite-based emissions estimates, typically estimates higher current emissions than the EPA which relies upon national reporting, accounting for the larger IEA values in several countries. However, for Uzbekistan and Russia, the IEA base emissions are much lower, at 670 and 13,600 kt yr −1 , respectively, versus 3000 and 24,800 kt yr −1 in the EPA analysis (Russian official reporting was revised downward since the EPA analysis).
Differences between cost estimates are more systematic across countries, with the IEA consistently much lower than EPA. Differences are linked to several factors, including the inclusion of “super-emitters” by the IEA, a scarcity of data on required capital and operational expenditures, and varying revenue assumptions and typical lifetimes for abatement measures (the EPA uses a 5% discount rate and the IEA 10%, which would generally lead to relatively lower costs for the EPA). For example, the EPA estimates incorporate uniform natural gas prices across segments, whereas the IEA has different prices for upstream and downstream segments. Mitigation measures also vary, with each having specific costs, revenue, and lifetime in both analyses.
For both gas and oil, IIASA analyses show much smaller mitigation potential for India than either the EPA or IEA analyses, whereas for China, the IIASA estimates lie between EPA and IEA values ( Table 2 ). For both countries, mitigation potentials vary by 300% to 600% across the three datasets for gas, oil, or oil plus gas—much larger than the 16% to 150% variations for coal. Turning to costs, IIASA analyses for gas and oil in India and China find large net revenues, whereas the IEA finds smaller revenues and EPA large net expenditures ( Table 2 ). IIASA’s lower costs are attributable to the lower discount rate (4%) that increases the value of future revenue from captured gas, as well as projecting increases in the value of future gas based on the IEA New Policies Scenario (whereas the IEA, for example, uses present-day prices as they examine immediate abatement).
The social cost of methane
The social cost of methane (SCM), monetizing climate change-related damages, has recently been reevaluated ( 114 ) based on results from three damage estimation models ( 115 – 117 ). Incorporating only the impacts of climate change, the SCM ranges from US$470–US$1700 tCH 4 −1 for 2020 across these models using 2.5% discounting (values in 2020 US$). The spread narrows greatly over time to US$1100–US$2300 in 2030 and US$2700–US$3700 in 2050. This indicates that the models differ greatly in their near-term climate damage while converging in their valuation of longer-term impact. The 2030 SCM is 8–15 times larger than the social cost of CO 2 in 2030 (with 2.5% discounting) using these models, a “global damage potential” much lower than metrics of 30 (GWP100) or 83 (GWP20) typically used to compare these gases. Using one of those same damage estimate models, as well as others, higher 2020 values were recently reported: US$2900 tCH 4 −1 for models using a stochastic rather than fixed discount rate by otherwise standard methods applying economic damage to current output and US$75,600 tCH 4 −1 using models applying damage to long-term economic growth which then compound over time ( 118 ). The latter not only dramatically boosts social costs but also global damage potential, which rises from 21 to 44 in their analysis.
These types of evaluations have inherent inconsistencies, however. They include the effects of methane-induced ozone changes on climate but not health. However, there is a robust evidence base for ozone-health impacts via methane photochemistry ( 4 , 77 , 78 , 119 – 121 ). Similarly, SCM estimates include the effects of climate and CO 2 exposure on ecosystems, including agriculture, but not ozone exposure ( 78 , 122 ). Several studies have evaluated the SCM accounting consistently for ozone damage. Based on adults-only health impacts with relatively weak ozone effects on cardiovascular-related deaths and incorporating climate-only valuations without compounding growth effects, they find substantially larger values of ~US$4300–US$4400 tCH 4 −1 for 2020 ( 4 , 78 ). Using both stronger cardiovascular impacts and impacts on children under 5 (Analysis E), those values rise to ~US$7000 tCH 4 −1 . Using either those values or the values incorporating economic growth impacts ( 118 ), virtually all current methane abatement options cost much less than the associated environmental damages.
Economic considerations, including profit versus abatement in oil production
Given that many low-cost controls are available, the imposition of even a modest price on methane emissions would incentivize some emission reductions and overcome implementation barriers based on marginal costs alone ( 3 ). Several examples of methane pricing exist: auctions under California’s emissions trading system in 2022 yielded prices of ~US$725 tCH 4 −1 ( 123 ), Norway has a US$1500 tCH 4 −1 fee on oil and gas operators, and the 2022 US Inflation Reduction Act sets a price on excess methane emissions from oil and gas of US$900 tCH 4 −1 in 2024, rising to US$1500 tCH 4 −1 after 2025. Under these types of pricing regimes, average abatement costs in most priority countries would become negative for coal ( Figure 10 ) and oil and gas ( Figure 8 ). Similarly, an International Monetary Fund (IMF) analysis recommends a rising price on methane reaching ~US$2100 tCH 4 −1 in 2030 to align emissions with the 2°C goal ( 124 ). A methane fee might be set to a politically practical value, the value needed to achieve a desired reduction (as in the IMF analysis), or the value of associated environmental damages (the SCM).
Economic analyses from a societal perspective, i.e., how a mitigation measure incurs costs and benefits for both public and private stakeholders (including long-term impacts on future generations), can help policymakers define emission reduction targets that aim to optimize welfare ( 125 ). Private-sector decision-makers have a different perspective, with higher discount rates and shorter return times on investments; mitigation measures generating net profits may sometimes be outcompeted by production activities generating even higher profits since capital is limited. The profit-maximizing investor will weigh the relative profits of possible investments and choose the one with the highest return, leaving investment opportunities with lower profits unfunded. Even mitigation costs without consideration of environmental impacts, as discussed here, can be misleading about private sector decision-making. For example, despite recent increases in gas prices resulting in increased profits from gas recovery during oil production, industry incentives to invest in this have weakened because the profit margin from oil production has increased more rapidly than that from extended gas recovery owing to an increasing spread between oil and gas prices.
To illustrate this, we compare returns from methane controls during oil production, such as the recovery of associated gas for reinjection or utilization and leak detection and repair programs, for two cases denoted “Jan 2020” and “July 2022” (Analysis I). These correspond approximately to global oil and gas markets in those months with historic lows and highs, respectively ( Table 3 ). When oil and gas prices are low, the two profit margins can overlap without a methane fee ( Figure 11 ). Under such conditions, methane recovery investments can be as or more profitable than investments in increased oil production. We then expect some voluntary investments into methane control without the introduction of legally binding regulations. As oil and gas prices climb to the July 2022 levels, the profit margin of increasing oil production quickly outpaces that of methane control without a fee. In an illustrative example of a US$1500 tonne −1 fee on methane, as in the US and Norway, methane abatement becomes generally more profitable than oil production with low prices, though this fee is sufficient to make only some abatement as profitable as production with high prices ( Figure 11 ).
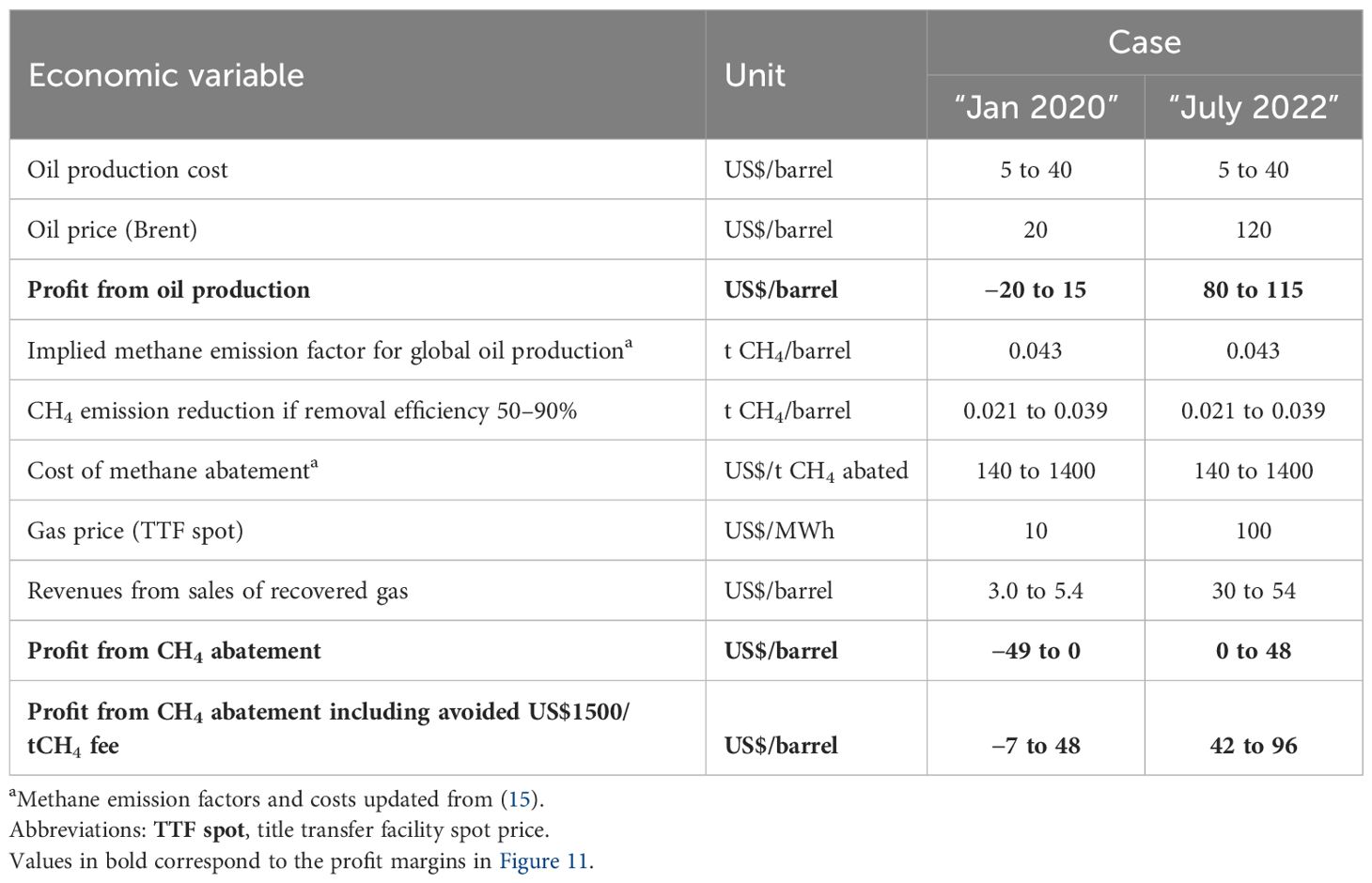
Table 3 Assumptions for the two fictive, illustrative cases “Jan 2020” and “July 2022”.
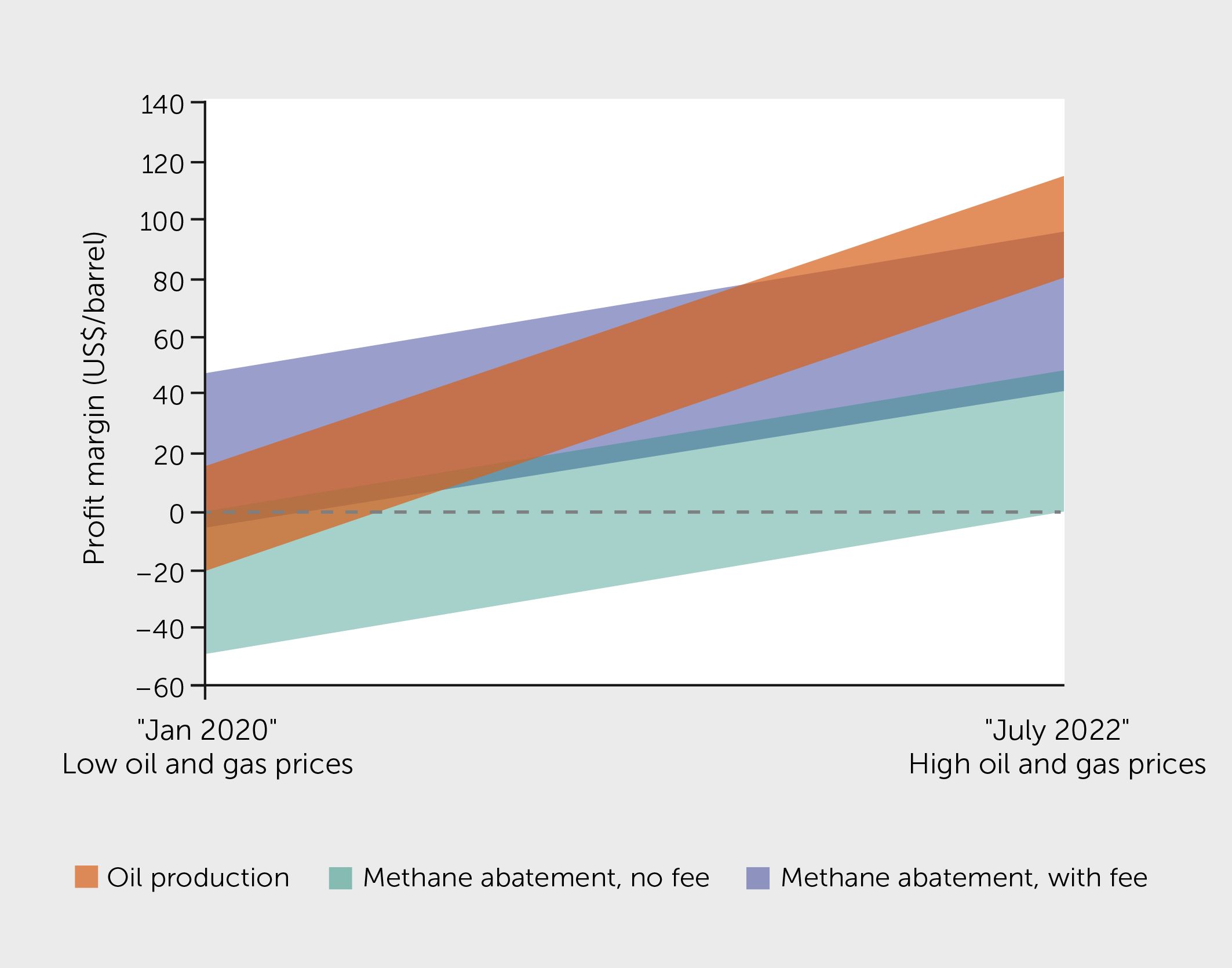
Figure 11 Variation in profit margins for oil production and methane abatement as fossil fuel prices change. Ranges for profit margins of oil production and methane abatement are shown for two illustrative cases “Jan 2020” and “July 2022” that correspond to historically low and high oil and gas prices, respectively (see Table 3 for assumptions). Profit margins for methane abatement are shown without a fee on emissions and with a US$1500 per tonne illustrative methane fee. See Methods (Analysis I) for further details.
This analysis helps explain the behavior of real-world markets, e.g., “Methane emissions remained stubbornly high in 2022 even as soaring energy prices made actions to reduce them cheaper than ever” ( 126 ). Profit-maximizing oil companies have a greater incentive to spend capital on increased production rather than voluntarily investing in methane control when prices are high, even though profits from such actions have increased. In such cases, oil companies can only be expected to invest in methane control if forced to do so through legally binding regulations. While actions to control methane from the fossil fuel sector entail substantial costs, the industry has ample resources compared with sectors such as waste or agriculture. For example, the IEA estimates that reducing energy-related methane emissions by 75% would require spending through 2030, which is <5% of the industry’s net 2023 income ( 127 ).
To reach abatement targets through private sector investments, policymakers need to ensure regulations are strong enough to overcome any competitive disadvantage of abatement investments relative to other operational investments. That measures are cost-effective from a societal perspective is no guarantee that abatement will happen without the introduction of additional regulations and policy incentives, such as requirements to use the best available technologies or a methane fee high enough to make abatement gains comparable to those available from new-source development from a private perspective ( Figure 11 ). The imperatives to both reduce methane rapidly this decade and transition to net zero CO 2 by the middle of the century imply that societies should consider granting companies social licenses to operate only if they are on course to both very low methane intensity by 2030 (including no routine venting or flaring) and to net zero CO 2 by 2050.
Conclusions and next steps
The GMP has created enormous policy momentum. Alongside it, the Global Methane Hub ( https://globalmethanehub.org/ ) links ~20 philanthropic organizations’ supporting action, and the CCAC links development banks with mitigation implementers. As such, there is an urgent need for expanded and improved knowledge of both the benefits of and opportunities for mitigation and access to finance to support the effective implementation of mitigation policies. This information can be provided with support tools that keep pace with rapidly advancing knowledge regarding current emission sources, especially via remote sensing.
Our analyses support three imperatives for methane mitigation. We illustrate how observations show increased methane concentration growth rates, which have recently reached the greatest values on record according to both ground-based and satellite data. Observed methane growth rates are now much higher than the mean predictions across models and far above levels consistent with Paris Climate Agreement goals. Human activities are predominantly responsible for the past ~15 years of growth—with contributions from increased emissions from wetlands due to anthropogenic global warming and from direct anthropogenic emissions. The first imperative is therefore to change course and reverse methane emission growth through stronger policy-led action targeting all major drivers of methane emissions as well as to greatly reduce CO 2 emissions rapidly.
The second imperative is to align methane and CO 2 mitigation. Major and rapid reductions in methane are integral to least-cost 1.5°C- and 2°C-consistent scenarios alongside the transformations needed to reach net zero CO 2 by ~2050. However, net zero methane emissions is not the target owing to abatement challenges for some sources and its short lifetime. Nevertheless, since methane and CO 2 each contribute to warming, maximizing reductions in methane emissions is important both for its own sake to ensure that 1.5°C- or 2°C-consistent CO 2 trajectories are feasible and to reduce CDR requirements. Methane and CO 2 mitigation actions are tightly interrelated: reducing methane emissions can directly contribute to reduced atmospheric CO 2 via carbon cycle interactions. Focusing on land use, we quantify how decreased livestock numbers afforded by reduced consumption of cattle-based foods not only help reduce methane emissions but also free up land to help meet projected needs for CDR at levels required to achieve long-term climate goals. Rapid and deep cuts to CO 2 and methane provide the strongest climate benefits across the century.
The third imperative highlights the need to optimize methane abatement policies. We show that both technological abatement options and systemic and behavioral choices must be addressed to reduce methane emissions. Our national-level analysis of methane mitigation opportunities highlights the need to address all subsectors when considering abatement options. We find that although many mitigation costs are low relative to real-world financial instruments and methane damage estimates, strong, legally binding regulations need to be in place even in the case of negative-cost options. To help policymakers and project funders, we created an online tool that explores different options and their cost-effectiveness. This tool supports policymakers by, for example, displaying (i) the most cost-effective options for countries to achieve a desired methane abatement objective economy-wide by sector or by subsector and (ii) the options in each country or countries that provide the largest abatement opportunities for a given spending level. Given substantial uncertainties in both emissions and costs, these data provide guidance for funders or policymakers who can then pursue more detailed studies. Funding equivalent to mitigation costs is not necessarily required since the cost analyses could support regulatory policies, e.g., by showing that they do not impose onerous burdens. For example, mitigation in the fossil sector is both large and low in cost in China and India, as are reductions in landfill methane in India, suggesting these two non-GMP countries have the potential to achieve major methane reductions with limited financial burdens.
The tool provides abatement potentials both as tonnes and percentages. The latter facilitates use with observations, for example, the identification of emission sources by satellites with global coverage but relatively low spatial resolution that are followed up by higher resolution site-specific quantification of emission rates ( Figure 12 ). These data will soon be complemented by the satellite missions Carbon Mapper, MethaneSAT, GOSAT-GW, Sentinel-5, and Satlantis as well as datasets produced by the Integrated Global Greenhouse Gas Information System and the International Methane Emissions Observatory. Automated reporting based on satellite observations promises to provide rapid information on emissions and progress in abatement [e.g., ( 49 ), ( 107 )] though updates to mitigation potentials and costs based on new data will take considerable time and effort.
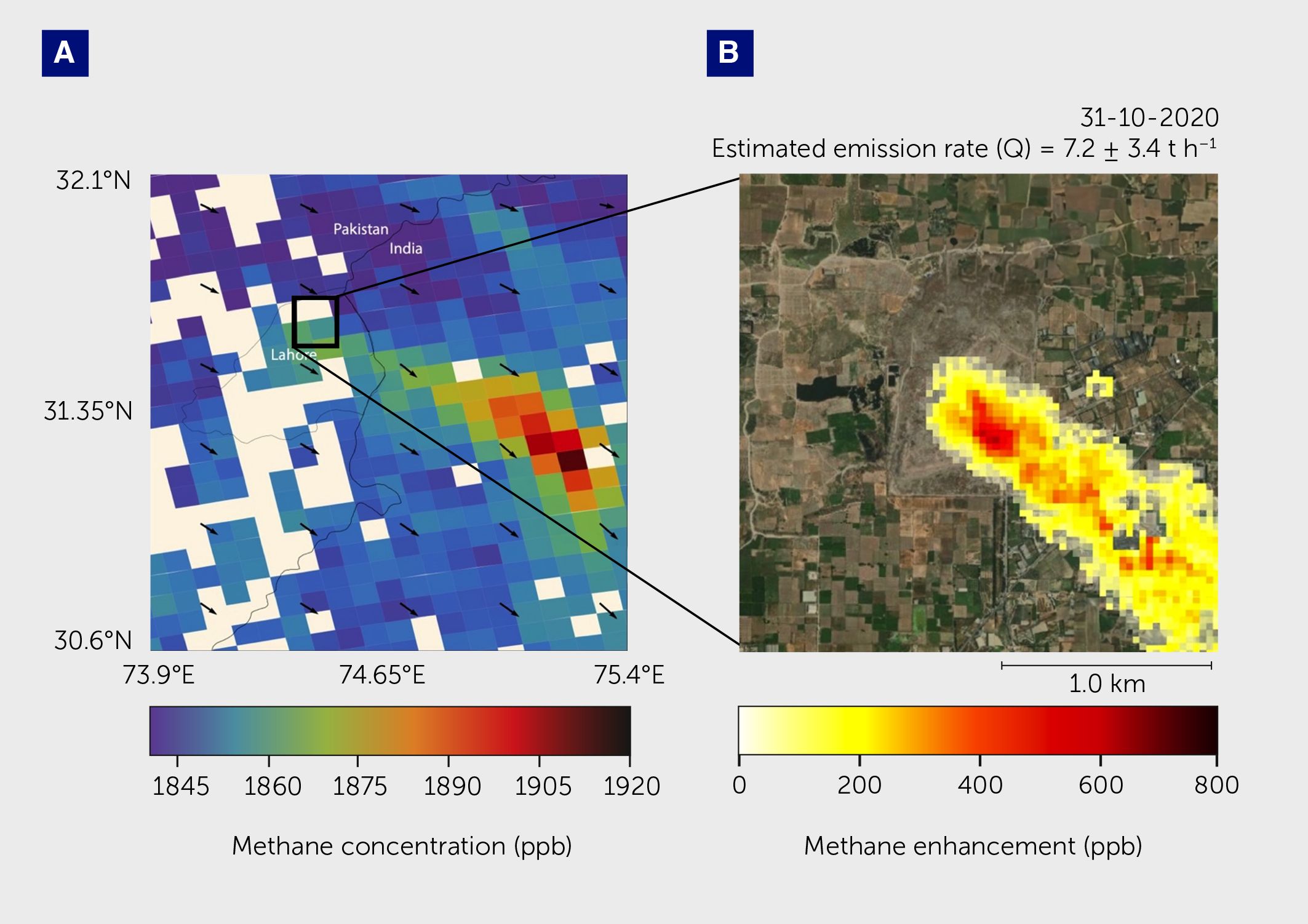
Figure 12 Example use of remote sensing to quantify methane emissions. (A) Methane observations from the TROPOMI instrument on 31 March 2019 over the region encompassing Lahore, Pakistan. (B) High-resolution measurement of methane enhancement over the northern part of the city observed by GHGSat on 31 October 2020. The emission source location matches the siting of the Lahore landfill, with Q indicating the estimated methane emission rate.
The new tool complements another showing the benefits of methane abatement ( http://shindellgroup.rc.duke.edu/apps/methane/ ). That tool allows the user to select global or regional methane mitigation options by sector and cost and then displays national-level benefits including ozone effects on human health, yields for several major staple crops, heat-related labor productivity, and the economic valuation of these.
Though methane has similar environmental impacts wherever it is emitted, co-emissions affect those living near sources with environmental justice implications [e.g., ( 128 , 129 )]. These include hazardous hydrocarbons, such as benzene, that are frequently emitted by gas and oil facilities, black carbon from flaring, and ammonia from manure ponds. Methane-producing infrastructure is often in areas with high social vulnerability [e.g., ( 130 )]. Accounting for co-emissions requires improved data on their spatial distribution and volume, especially in areas with nearby vulnerable populations.
There is also a need to improve understanding of several physical processes influencing the climate impacts of methane emissions. Methane-induced ozone increases affect the carbon cycle, amplifying the climate impact of methane, but the magnitude of this effect is highly uncertain ( 12 ). Additionally, methane affects particle formation via oxidants, producing aerosol-cloud interactions that may augment the climate impact of methane ( 131 ). Studies also report divergent results for the net cloud response to methane when the shortwave absorption of methane is accounted for ( 132 , 133 ). A better understanding of the response of natural methane emissions to climate change is also needed. Improved capabilities to monitor emissions from difficult-to-access methane-source areas (e.g., wetlands) using remote sensing should help constrain changes in natural sources over the coming decade. A research agenda for methane removal technologies, which could be deployed in the unlikely event of a surge in natural emissions, has been called for [e.g., ( 134 )] and is currently being assessed ( https://www.nationalacademies.org/our-work/atmospheric-methane-removal-development-of-a-research-agenda ).
Though additional observations and improved scientific understanding will be valuable, securing the benefits for climate, health, labor productivity, and crops ( 4 , 79 ) that are the rationale for the GMP requires immediate implementation to achieve the emission reductions envisioned by 2030. Not only is our understanding of methane science and mitigation options sufficient to act upon, but political support is evidenced by the GMP, and financial support is growing. It is also becoming clearer how methane fees would achieve climate goals and enhance well-being. In the face of ever-increasing climate damages, including heat waves, flooding, storms, and fires, the world has a real opportunity to reduce the rate at which these effects grow between now and 2050 via methane action, with the main impediment being the will to implement the known solutions.
Analysis A: methane growth/emissions vs projections
Methane abundance growth rates during the 2020s are taken from “no climate policy” baseline scenarios from several recent multi-model intercomparison projects using integrated assessment models: ADVANCE ( https://www.fp7-advance.eu/ ), NAVIGATE ( https://www.navigate-h2020.eu/ ) ( 14 ), and ENGAGE ( https://www.engage-climate.org/ ). NAVIGATE and ENGAGE scenarios are the most recent and include updates to actual trends in energy demand, costs, etc., and legislation through ~2020. This dataset includes results from the following IAMs: AIM/CGE 2.0, IMAGE (versions 3.0.1, 3.0.2, and 3.2), MESSAGE-GLOBIOM 1.0, MESSAGEix-GLOBIOM 1.1, POLES, REMIND 1.7, REMIND-MAgPIE (versions 1.5, 2.0–4.1, and 2.1–4.2), WITCH 5.0, and WITCH- GLOBIOM 4.2.
Baseline projections are also included from two “bottom-up” analyses by the International Institute for IIASA ( 15 ) and the EPA ( 16 ). The IIASA analysis uses their Greenhouse gas and Air pollution Interactions and Synergies (GAINS) model in which baseline emission estimates reflect expected impacts on emissions from current legislation to control emissions. Future methane emissions in GAINS by 2050 are developed based on macroeconomic and energy sector activity drivers from the IEA World Energy Outlook 2018 New Policies Scenario ( 135 ), agricultural sector activity drivers from the Food and Agricultural Organisation of the United Nations (FAO) ( 136 ), and IIASA’s own projections of solid waste and wastewater generation consistent with their relevant macroeconomic drivers. By incorporating policies projected forward by the IEA in 2018 in the energy scenario, these projections are expected to be similar to the NAVIGATE and ENGAGE baselines. The EPA’s projections are based on projected changes in underlying drivers taken from various globally available activity data sources depending on the source category. Trends in energy production and consumption are based on the United States Energy Information Administration 2017 International Energy Outlook Reference Case scenario. Growth rates in crop and livestock production are from International Food Policy Research Institute’s IMPACT model (International Model for Policy Analysis of Agricultural Commodities and Trade) ( 137 ). The full methodology is discussed in the documentation accompanying the EPA’s Global non-CO 2 greenhouse gas emission projections & mitigation report ( 16 ). Neither the integrated assessment models nor the bottom-up analyses include changes in natural methane emissions.
A simple box model with a sink proportional to the atmospheric abundance of methane is used both to derive emission and sink estimates ( Figure 1 ) and to convert scenario emissions to estimated concentration changes ( Figure 2 ). The atmospheric residence time for methane is 9.1 years for 2020 methane concentrations in this model, consistent with the value reported in the IPCC AR6 ( 12 ).
Analysis B: projected methane emissions reductions under 1.5°C-consistent scenarios
This analysis utilizes the scenario dataset analyzed in the IPCC AR6 ( 59 ). We include all scenarios classified as being below 1.5°C in 2100 (>50% probability) with either no or limited overshoot and for which agricultural as well as total methane emissions were available. There are 53 scenarios from eight models that represent five separate model families: AIM/CGE 2.2 and AIM/Hub-Global 2.0; IMAGE 3.2; MESSAGE-GLOBIOM 1.1; REMIND 2.1, REMIND-MAgPIE 2.1–4.2 and 2.1–4.3; and WITCH 5.0. Data were obtained from the AR6 Scenario Database ( 86 ), release 1.1.
Analysis C: connection between land area use for BECCS and pasture
This analysis utilizes two sets of scenarios from the AR6 scenario database ( 86 ). We examine the relationship between the deployment of BECCS and the area used for pasture (area used for fodder was not available) using scenarios classified as keeping warming below 1.5°C with limited or no overshoot as well as those keeping warming below 1.5°C with high overshoot. The latter are included to obtain a larger sample of models given substantial intermodal variability in estimates of future BECCS deployment. Results are available from seven model families: AIM, GCAM, IMAGE, MESSAGE, REMIND, COFFEE, and WITCH. From these scenarios, we also analyze decadal changes in the multi-model means and individual scenarios for these two quantities from the 2040s (or 2030s) to 2090s.
A second set of scenarios is used to explore how land use trade-offs including land area used for afforestation vary across IAMs. We use an expanded set of scenarios classified as under 2°C as afforestation diagnostics were not available from as many models. Even using this larger dataset, we found only eight models that provided all the required outputs. As this analysis compares land used for carbon uptake (afforestation and bioenergy crops) with pasture area across multiple scenarios within a single model, we excluded three models that had six or fewer scenarios. One additional model, a variant of REMIND, has minimal changes in land deployed for carbon uptake so does not provide useful input for this analysis (though averages and ranges are not sensitive to the inclusion of that model). For the remaining four models (IMAGE 3.2, MESSAGEix-GLOBIOM 1.1, REMIND-MAgPIE 1.7–3.0, and WITCH 5.0), 22–106 scenarios were available (206 in total), allowing a robust characterization of the land use relationship for each of these models. In this analysis, afforestation is converted from the reported value in tCO 2 to area using 12 tCO 2 per ha ( 138 ).
Analysis D: climate impact of decarbonization and methane reduction
We analyzed the response of global mean annual average surface air temperatures to emissions under various scenarios to isolate the effects of decarbonization and targeted methane emission controls. The emissions scenarios are based upon the SSPs, using averages across 1.5°C scenarios (nominal 1.9 W m −2 forcing in 2100) under SSPs 1, 2, and 5 as 1.5°C was infeasible under SSP3 in four of four models and under SSP4 in two of three models. From those scenarios, we separate the effects of decarbonization from targeted methane abatement based on the methane abatement associated with decreasing fossil fuel use ( 4 , 82 , 95 ), which is classified as part of decarbonization, relative to all other methane reductions, which includes the remaining portion of fossil fuel-sector methane abatement and all methane abatement in the agriculture and waste sectors.
Temperature responses to those emissions relative to constant 2020 emissions were calculated using absolute global temperature potentials (AGTPs), as in prior work ( 4 , 66 , 78 ). The yearly AGTPs represent the global mean temperature change per kilogram of emission each year after those emissions based on an impulse-response function for the climate system, as is used in IPCC reports for selected example years, e.g., AGTP50 or AGTP100 ( 69 ). This analysis relies on AGTPs created using the transient climate response averaged over the last generation of climate models (CMIP5) ( 139 ), which is very similar to that reported from the latest generation ( 63 ). The response to methane is calibrated to match the global mean annual average temperature response from the full composition-climate models reported in the Global Methane Assessment’s climate simulations ( 4 ).
Analysis E: impact of methane abatement on temperature and health
This analysis presents global mean annual average temperature responses using the same methodology as Analysis D but in this case applied to scenarios based upon baseline and 1.5°C-consistent scenarios under the SSP2 pathway. SSP2 is chosen as it lies in the middle of the three for which models produced several 1.5°C consistent scenarios (SSPs 1, 2, and 5), consistent with its “middle-of-the-road” narrative description ( 96 ).
This analysis also presents health impacts based on changes in exposure to surface ozone. The GMA used five global composition-climate models to evaluate the effect of methane emissions on the maximum daily 8-hour ozone exposure averaged over the year (MDA8-annual). This was the metric most closely linked to increases in premature deaths from ozone in one of the largest epidemiological studies to date ( 140 ) as well as in a second large United States study that obtained very similar exposure-response results ( 141 ). This analysis utilizes the multi-model mean changes in this metric per unit methane emission change to derive the effect on human health due to reduced risk of both respiratory and cardiovascular premature mortality with decreasing ozone exposure.
We note that groups such as the EPA and Global Burden of Disease (GBD) do not include ozone-related cardiovascular premature deaths—the EPA’s expert panel reports that “evidence for long-term ozone exposure and cardiovascular effects is suggestive of, but insufficient to infer, a causal relationship” ( 142 ). However, a recent cohort study in China ( 143 ) reports a strong relationship and a much higher risk increment per unit exposure than that used here based on the United States studies. To characterize the range of potential methane-ozone-health impacts, we also evaluated the maximum daily 8-hour ozone exposure averaged over the 6-month period of maximum exposures (MDA8–6mon), the metric used in the Chinese epidemiological analysis. We apply the exposure–response relationship for cardiovascular disease of Niu et al. ( 143 ) using the same theoretical minimum risk exposure level (a threshold) as in the United States study [26.7 ppb ( 140 )], as this value is below any exposures in Niu et al. The results are only modestly sensitive to the use of this threshold, however, with values ~20% less without the threshold, well within uncertainty ranges. We find 1930 [1110–2510: 95% confidence interval (CI)] deaths per Mt methane emission based on the exposure-response of Niu et al. ( 143 ), a best estimate value much larger than even the high end of the 690 (210–1120: 95% CI) deaths per MtCH 4 found using the Turner et al. ( 140 ) relationship ( 4 ). Note that another large Chinese cohort study ( 144 ) reported more than double the increased risk of cardiovascular death due to increased ozone exposure relative to Niu et al. ( 143 ), suggesting that even our high-end estimate could be substantially too small.
In addition to the differing estimates of the effect of ozone on premature cardiovascular deaths, another recent analysis reports a strong relationship between ozone exposure and increased premature death in children aged under 5 years in low- and middle-income countries ( 145 ). Such an effect would be distinct from other effects analyzed here as the other studies included only populations aged 18 and older ( 143 ) or 30 and older ( 140 ). The impacts on children aged 0 to 5 were reported in response to MDA8–6mon, and we used this metric to again evaluate the effects of changing methane emissions for ozone exposures above 51 ppb, as reported in the epidemiological study. We find an additional 320 (125–485: 95% CI) premature deaths in children under 5.
Combining the 740 (460–990) adult respiratory deaths ( 4 ) with the adult cardiovascular deaths found here based on the Chinese cohort ( 143 ) and the under-5 age group deaths gives a total value of 3000 (2100–3600) per MtCH 4 . Using standard valuation methods ( 4 ), this leads to a valuation of US$5200 (3650–6250) per tCH 4 .
Human health impacts were calculated using 2015 population data from the Gridded Population of the World (GPW) version 4 ( 146 ) and 2015 baseline mortality rates from the GBD project ( 147 ) for each country of the world.
Analysis F: impact of methane abatement on heavy/outdoor labor
We assess the effects of changes in heat exposure due to mitigation of methane emissions on potential labor productivity within the heavy labor category, which primarily includes outdoor workers in agriculture, forestry and fisheries, and construction ( 100 ). The effects of methane abatement are evaluated relative to a “middle-of-the-road” SSP2 scenario, as in Analysis E. Uncertainties are characterized using multiple impact functions, namely those of Kjellstrom et al. ( 148 ), Foster et al. ( 149 ), and the International Organization for Standardization (ISO) Standard 7243 ( 150 ), using the approach of Bröde et al. ( 151 ). Analyses are performed for both the case of workers in the sun and in the shade.
Valuation of the avoided labor losses uses estimates from the International Labour Organization (ILO) of the fraction of the overall working-age population (ages 15–64) in each country that works in heavy labor ( 152 ), multiplied by the spatially gridded population ages 15–64 [Gridded Population of the World v4 data ( 146 )] to estimate the number of workers in a given category and their spatial distribution. We then overlay the heavy labor hours lost by these workers to obtain total hours lost. We next calculate average value added per worker in agriculture, forestry and fisheries, and construction by dividing the total value added in 2017 ( 153 ) by the total working-age employment in a given category. This is then converted to value per hour assuming a 12-hour workday and 365 days/year (a maximalist assumption, though common in the labor economics literature, so the value of hours lost reported here is conservative). We then multiply the hourly value added per worker by the heavy labor hours lost to estimate the economic costs of heat-related productivity losses. Finally, values are converted from 2017 local currency units (LCU) to 2017 PPP-adjusted international dollars (2017 PPP$) by dividing a country’s LCU by its gross domestic product 2017 PPP conversion rate (LCU/US$). We sum the losses over all countries (n=163) to obtain the estimated global output loss.
Analysis G and H: national-level methane mitigation analysis of abatement potentials and costs
National mitigation potentials and their associated costs are evaluated primarily based on the data from the EPA ( 16 ) and from the IEA ( 90 ). The EPA data cover all sectors and include projected changes in both baseline emissions and mitigation. Mitigation potentials change over time due to factors such as projected technology turnover and improvements in technology over time. Potentials are estimated through 2050 and use a discount rate of 5% in cost estimates (e.g., for the value of captured gas). The IEA analysis includes only the fossil fuel sector and analyzes present-day abatement potentials associated with targeted control measures. This analysis uses a discount rate of 10% in its cost estimates.
Limited national data are also included from an analysis by IIASA, though this analysis is primarily done at the regional level ( 15 ). As with the EPA analysis, these mitigation potentials and costs cover all sectors and include time-dependent estimates of both changes in baseline emissions and mitigation. The latter include sector-specific assumptions about technology turnover times, based on the literature, improvements in technology over time, and the achievable pace of regulations. This analysis includes discount rates of 4% and 10% in their cost evaluation and also extends to 2050. EPA and IIASA data are evaluated for 2030 whereas IEA estimates are for 2022.
As presented in the main text, abatement options have been grouped into functionally similar categories to facilitate readability and allow comparison across estimates. An online tool facilitating analysis of the national level EPA and IEA has been created that allows users to sort the available national abatement options by sector according to their costs. The user can specify either a mitigation target or a spending target and can also compare across the EPA and IEA datasets (within the fossil fuel sector) and countries. The tool is available at https://github.com/psadavarte/Methane_mitigation_webtool .
Analysis I: profit/return from controlling methane emissions versus price (oil production)
To examine the implications of price fluctuations on oil companies’ incentives to invest in methane abatement, we compared two fictive cases called “Jan 2020” and “July 2022”. These approximate the situations in the global oil and gas markets in January 2020, when the world oil and gas prices stood at a historical low at ~US$20/barrel for oil (Brent) and about ~US$10/MWh for gas (title transfer facility [TTF] spot price), and July 2022, when the same prices stood at a historic high at about US$120/barrel for oil and US$100/MWh for gas. Our analysis assumes that there are no appreciable changes in the costs of oil production or methane abatement, the impact factors (methane released per barrel) for oil-related methane emissions, or the effectiveness of methane abatement to isolate the effects of commodity price changes.
Supplementary material
The Supplementary Material for this article can be found online at: https://www.frontiersin.org/articles/10.3389/fsci.2024.1349770/full#supplementary-material
Acknowledgments
We thank Katie Owens for analyses of AR6 scenarios and the Global Methane Hub for financial support.
Author contributions
DS: Conceptualization, Funding acquisition, Investigation, Supervision, Writing – original draft. PS: Investigation, Writing – review & editing. IA: Investigation, Visualization, Writing – review & editing. TB: Investigation, Writing – review & editing. GD: Writing – original draft. LH-I: Conceptualization, Investigation, Writing – original draft. BP: Investigation, Writing – review & editing. MS: Investigation, Writing – review & editing. GS: Investigation, Writing – review & editing. SS: Investigation, Writing – review & editing. KR: Data curation, Investigation, Visualization, Writing – review & editing. LP: Investigation, Writing – review & editing. ZQ: Writing – review & editing. GF: Writing – review & editing, Formal Analysis. JM: Investigation, Visualization, Writing – review & editing.
Data availability statement
The original contributions presented in the study are included in the article/ Supplementary Material . Further inquiries can be directed to the corresponding author.
The author(s) declare financial support was received for the research, authorship, and/or publication of this article. This work was funded by the Global Methane Hub through Windward Fund Grant 016011-2022-01-01 and through the European Union FOCI program. The funders had no role in the study design; in the collection, analysis, and interpretation of data; in the writing of the report; and in the decision to submit the paper for publication.
Conflict of interest
The authors declare that the research was conducted in the absence of financial relationships that could be construed as a potential conflict of interest.
The reviewer FOC declared a past co-authorship with the author SS to the handling editor.
Publisher’s note
All claims expressed in this article are solely those of the authors and do not necessarily represent those of their affiliated organizations, or those of the publisher, the editors and the reviewers. Any product that may be evaluated in this article, or claim that may be made by its manufacturer, is not guaranteed or endorsed by the publisher.
1. Masson-Delmotte V, Zhai AP, Connors SL, Péan C, Berger S, Caud N, et al, editors. Climate Change 2021: The Physical Science Basis. Contribution of Working Group I to the Sixth Assessment Report of the Intergovernmental Panel on Climate Change . Cambridge: Cambridge University Press (2021). doi: 10.1017/9781009157896
CrossRef Full Text | Google Scholar
2. Rosane P, Naran B, Ortega Pastor A, Conolly J, Wignarajah D. The landscape of methane abatement finance . Climate policy initiative (2022). Available at: https://www.climatepolicyinitiative.org/publication/the-landscape-of-methane-abatement-finance/ .
Google Scholar
3. Olczak M, Piebalgs A, Balcombe P. A global review of methane policies reveals that only 13% of emissions are covered with unclear effectiveness. One Earth (2023) 6(5):519–35. doi: 10.1016/j.oneear.2023.04.009
4. United Nations Environment Programme and Climate and Clean Air Coalition. Global Methane Assessment: Benefits and Costs of Mitigating Methane Emissions . Nairobi: UNEP (2021). Available at: https://www.unep.org/resources/report/global-methane-assessment-benefits-and-costs-mitigating-methane-emissions .
5. International Energy Agency. World Energy Outlook (2021) Paris: IEA. Available at: https://www.iea.org/reports/world-energy-outlook-2021/keeping-the-door-to-15–0c-open .
6. Shukla PR, Skea J, Slade R, Al Khourdajie R, van Diemen D, McCollum M, et al, editors. Climate Change 2022: Mitigation of Climate Change. Contribution of Working Group III to the Sixth Assessment Report of the Intergovernmental Panel on Climate Change . Cambridge: Cambridge University Press (2022). doi: 10.1017/9781009157926
7. Nisbet EG, Manning MR, Dlugokencky EJ, Fisher RE, Lowry D, Michel SE, et al. Very strong atmospheric methane growth in the 4 years 2014–2017: implications for the Paris Agreement. Global Biogeochem Cycles (2019) 33(3):318–42. doi: 10.1029/2018GB006009
8. Nisbet EG, Manning MR, Dlugokencky EJ, Michel SE, Lan X, Röckmann T, et al. Atmospheric methane: comparison between methane’s record in 2006–2022 and during glacial terminations. Global Biogeochem Cycles (2023) 37(8):e2023GB007875. doi: 10.1029/2023GB007875
9. Lan X, Thoning KW, Dlugokencky EJ. Trends in globally-averaged CH 4 , N 2 O, and SF 6 determined from NOAA Global Monitoring Laboratory measurements. Version 2024–04. National Oceanic and Atmospheric Administration Global Monitoring Laboratory (2022). doi: 10.15138/P8XG-AA10.
10. World Meteorological Organization. WMO Greenhouse Gas Bulletin No. 19 – 15 November 2023. The State of Greenhouse Gases in the Atmosphere Based on Global Observations through 2022 . Geneva: WMO (2023). Available at: https://library.wmo.int/idurl/4/68532 .
11. Copernicus Atmospheric Monitoring Service. Greenhouse gas concentrations (2023). Available at: https://climate.copernicus.eu/climate-indicators/greenhouse-gas-concentrations .
12. Szopa S, Naik V, Adhikary B, Artaxo P, Berntsen T, Collins WD, et al. Short-lived climate forcers. In: Masson-Delmotte V, Zhai P, Pirani A, Connors SL, Péan C, Berger S, et al, editors. Climate Change 2021: the Physical Science Basis. Contribution of Working Group I to the Sixth Assessment Report of the Intergovernmental Panel on Climate Change . Cambridge: Cambridge University Press (2021) 817–922. doi: 10.1017/9781009157896.008
13. United Nations Environment Programme and Climate and Clean Air Coalition. Global Methane Assessment 2030 Baseline Report . Nairobi: UNEP (2022), Available at: https://www.unep.org/resources/report/global-methane-assessment-2030-baseline-report .
14. Harmsen M, Kriegler E, Van Vuuren DP, van der Wijst KI, Luderer G, Cui R, et al. Integrated assessment model diagnostics: key indicators and model evolution. Environ Res Lett (2021) 16(5):054046. doi: 10.1088/1748-9326/abf964
15. Höglund-Isaksson L, Gómez-Sanabria A, Klimont Z, Rafaj P, Schöpp W. Technical potentials and costs for reducing global anthropogenic methane emissions in the 2050 timeframe – results from the GAINS model. Environ Res Commun (2020) 2(2):025004, Available at: https://iopscience.iop.org/article/10.1088/2515-7620/ab7457 . doi: 10.1088/2515-7620/ab7457
16. United States Environmental Protection Agency. Global Non-CO 2 Greenhouse Gas Emission Projections & Mitigation [EPA-430-R-19-010] . Washington, DC: U.S. EPA (2019). Available at: https://www.epa.gov/sites/default/files/2019-09/documents/epa_non-co2_greenhouse_gases_rpt-epa430r19010.pdf .
17. Rogelj J, Shindell D, Jiang K, Fifita S, Forster P, Ginzburg V, et al. Mitigation pathways compatible with 1.5°C in the context of sustainable development. In: Masson-Delmotte V, Zhai P, Pörtner HO, Roberts D, Skea J, Shukla PR, et al, editors. Special Report on Global Warming of 1.5°C, Intergovernmental Panel on Climate Change . Cambridge: Cambridge University Press (2018) 93–174. doi: 10.1017/9781009157940.004
18. Jackson RB, Saunois M, Bousquet P, Canadell JG, Poulter B, Stavert AR, et al. Increasing anthropogenic methane emissions arise equally from agricultural and fossil fuel sources. Environ Res Lett (2020) 15(7):071002. doi: 10.1088/1748-9326/ab9ed2
19. Canadell JG, Monteiro PMS, Costa MH, Cotrim da Cunha L, Cox PM, Eliseev AV, et al. Global carbon and other biogeochemical cycles and feedbacks. In: Masson-Delmotte V, Zhai P, Pirani A, Connors SL, Péan C, Berger S, et al, editors. Climate Change 2021: The Physical Science Basis. Contribution of Working Group I to the Sixth Assessment Report of the Intergovernmental Panel on Climate Change . Cambridge: Cambridge University Press (2021) 673–816. doi: 10.1017/9781009157896.007
20. Zhang Z, Poulter B, Knox S, Stavert A, McNicol G, Fluet-Chouinard E, et al. Anthropogenic emission is the main contributor to the rise of atmospheric methane during 1993–2017. Natl Sci Rev (2022) 9(5):nwab200. doi: 10.1093/nsr/nwab200
PubMed Abstract | CrossRef Full Text | Google Scholar
21. Thanwerdas J, Saunois M, Berchet A, Pison I, Bousquet P. Investigation of the renewed methane growth post-2007 with high-resolution 3-D variational inverse modeling and isotopic constraints. Atmos Chem Phys (2024) 24(4):2129–67. doi: 10.5194/acp-24-2129-2024
22. Yin Y, Chevallier F, Ciais P, Bousquet P, Saunois M, Zheng B, et al. Accelerating methane growth rate from 2010 to 2017: leading contributions from the tropics and East Asia. Atmos Chem Phys (2021) 21(16):12631–47. doi: 10.5194/acp-21-12631-2021
23. Feng L, Palmer PI, Zhu S, Parker RJ, Liu Y. Tropical methane emissions explain large fraction of recent changes in global atmospheric methane growth rate. Nat Commun (2022) 13(1):1378. doi: 10.1038/s41467-022-28989-z
24. Oh Y, Zhuang Q, Welp LR, Liu L, Lan X, Basu S, et al. Improved global wetland carbon isotopic signatures support post-2006 microbial methane emission increase. Commun Earth Environ (2022) 3(1):1–12. doi: 10.1038/s43247-022-00488-5
25. Zhang Z, Poulter B, Feldman AF, Ying Q, Ciais P, Peng S, et al. Recent intensification of wetland methane feedback. Nat Clim Change (2023) 13(5):430–3. doi: 10.1038/s41558-023-01629-0
26. Gedney N, Huntingford C, Comyn-Platt E, Wiltshire A. Significant feedbacks of wetland methane release on climate change and the causes of their uncertainty. Environ Res Lett (2019) 14(8):84027. doi: 10.1088/1748-9326/ab2726
27. Thornhill G, Collins W, Olivié D, Skeie RB, Archibald A, Bauer S, et al. Climate-driven chemistry and aerosol feedbacks in CMIP6 Earth system models. Atmos Chem Phys (2021) 21(2):1105–26. doi: 10.5194/acp-21-1105-2021
28. Kleinen T, Gromov S, Steil B, Brovkin V. Atmospheric methane underestimated in future climate projections. Environ Res Lett (2021) 16(9):094006. doi: 10.1088/1748-9326/ac1814
29. Cheng CH, Redfern SAT. Impact of interannual and multidecadal trends on methane-climate feedbacks and sensitivity. Nat Commun (2022) 13(1):3592. doi: 10.1038/s41467-022-31345-w
30. Zhang Z, Zimmermann NE, Calle L, Hurtt G, Chatterjee A, Poulter B. Enhanced response of global wetland methane emissions to the 2015–2016 El Niño-Southern Oscillation event. Environ Res Lett (2018) 13(7):074009. doi: 10.1088/1748-9326/aac939
31. Froitzheim N, Majka J, Zastrozhnov D. Methane release from carbonate rock formations in the Siberian permafrost area during and after the 2020 heat wave. Proc Natl Acad Sci USA (2021) 118(32):e2107632118. doi: 10.1073/pnas.2107632118
32. Kleber GE, Hodson AJ, Magerl L, Mannerfelt ES, Bradbury HJ, Zhu Y, et al. Groundwater springs formed during glacial retreat are a large source of methane in the high Arctic. Nat Geosci (2023) 16(7):597–604. doi: 10.1038/s41561-023-01210-6
33. Chen Z, Balasus N, Lin H, Nesser H, Jacob DJ. African rice cultivation linked to rising methane. Nat Clim Change (2024) 14(2):148–51. doi: 10.1038/s41558-023-01907-x
34. Laughner JL, Neu JL, Schimel D, Wennberg PO, Barsanti K, Bowman KW, et al. Societal shifts due to COVID-19 reveal large-scale complexities and feedbacks between atmospheric chemistry and climate change. Proc Natl Acad Sci USA (2021) 118(46):e2109481118. doi: 10.1073/pnas.2109481118
35. Peng S, Lin X, Thompson RL, Xi Y, Liu G, Hauglustaine D, et al. Wetland emission and atmospheric sink changes explain methane growth in 2020. Nature (2022) 612(7940):477–82. doi: 10.1038/s41586-022-05447-w
36. Stevenson DS, Derwent RG, Wild O, Collins WJ. COVID-19 lockdown emission reductions have the potential to explain over half of the coincident increase in global atmospheric methane. Atmos Chem Phys (2022) 22(21):14243–52. doi: 10.5194/acp-22-14243-2022
37. Qu Z, Jacob DJ, Zhang Y, Shen L, Varon DJ, Lu X, et al. Attribution of the 2020 surge in atmospheric methane by inverse analysis of GOSAT observations. Environ Res Lett (2022) 17(9):094003. doi: 10.1088/1748-9326/ac8754
38. Feng L, Palmer PI, Parker RJ, Lunt MF, Bösch H. Methane emissions are predominantly responsible for record-breaking atmospheric methane growth rates in 2020 and 2021. Atmos Chem Phys (2023) 23(8):4863–80. doi: 10.5194/acp-23-4863-2023
39. Food and Agriculture Organization of the United Nations. FAOSTAT Online Statistical Service (2024). Available at: https://www.fao.org/statistics/en/ .
40. CNBC. Mexico’s state oil company illegally flared more than US$342 million worth of hydrocarbons. CNBC (2023). Available at: https://www.cnbc.com/2023/01/18/Mexicos-pemex-illegally-flared-over-342-million-worth-of-hydrocarbons.html .
41. Lyon DR, Hmiel B, Gautam R, Omara M, Roberts KA, Barkley ZR, et al. Concurrent variation in oil and gas methane emissions and oil price during the COVID-19 pandemic. Atmos Chem Phys (2021) 21(9):6605–26. doi: 10.5194/acp-21-6605-2021
42. Plant G, Kort EA, Brandt AR, Chen Y, Fordice G, Gorchov Negron AM, et al. Inefficient and unlit natural gas flares both emit large quantities of methane. Science (2022) 377(6614):1566–71. doi: 10.1126/science.abq0385
43. Irakulis-Loitxate I, Guanter L, Maasakkers JD, Zavala-Araiza D, Aben I. Satellites detect abatable super-emissions in one of the world’s largest methane hotspot regions. Environ Sci Technol (2022) 56(4):2143–52. doi: 10.1021/acs.est.1c04873
44. Deng Z, Ciais P, Tzompa-Sosa ZA, Saunois M, Qiu C, Tan C, et al. Comparing national greenhouse gas budgets reported in UNFCCC inventories against atmospheric inversions. Earth Syst Sci Data (2022) 14(4):1639–75. doi: 10.5194/essd-14-1639-2022
45. Shen L, Gautam R, Omara M, Zavala-Araiza D, Maasakkers JD, Scarpelli TR, et al. Satellite quantification of oil and natural gas methane emissions in the US and Canada including contributions from individual basins. Atmos Chem Phys (2022) 22(17):11203–15. doi: 10.5194/acp-22-11203-2022
46. Sherwin ED, Rutherford JS, Zhang Z, Chen Y, Wetherley EB, Yakovlev PV, et al. US oil and gas system emissions from nearly one million aerial site measurements. Nature (2024) 627(8003):328–34. doi: 10.1038/s41586-024-07117-5
47. Duren RM, Thorpe AK, Foster KT, Rafiq T, Hopkins FM, Yadav V, et al. California’s methane super-emitters. Nature (2019) 575(7781):180–4. doi: 10.1038/s41586-019-1720-3
48. Lauvaux T, Giron C, Mazzolini M, d’Aspremont A, Duren R, Cusworth D, et al. Global assessment of oil and gas methane ultra-emitters. Science (2022) 375(6580):557–61. doi: 10.1126/science.abj4351
49. Schuit BJ, Maasakkers JD, Bijl P, Mahapatra G, Van den Berg A-W, Pandey S, et al. Automated detection and monitoring of methane super-emitters using satellite data. Atmos Chem Phys (2023) 23(16):9071–98. doi: 10.5194/acp-23-9071-2023
50. McNorton J, Bousserez N, Agustí-Panareda A, Balsamo G, Cantarello L, Engelen R, et al. Quantification of methane emissions from hotspots and during COVID-19 using a global atmospheric inversion. Atmos Chem Phys (2022) 22(9):5961–81. doi: 10.5194/acp-22-5961-2022
51. International Energy Agency. World Energy Investment 2022 . Paris: IEA (2022). Available at: https://www.iea.org/reports/world-energy-investment-2022 .
52. Oh Y, Bruhwiler L, Lan X, Basu S, Schuldt K, Thoning K, et al. CarbonTracker CH4 2023 . National Oceanic and Atmospheric Administration Global Monitoring Laboratory (2023). doi: 10.25925/40JT-QD67
53. Hodson EL, Poulter B, Zimmermann NE, Prigent C, Kaplan JO. The El Niño–Southern Oscillation and wetland methane interannual variability. Geophys Res Lett (2011) 38(8):L08810. doi: 10.1029/2011GL046861
54. Zhu Q, Peng C, Ciais P, Jiang H, Liu J, Bousquet P, et al. Interannual variation in methane emissions from tropical wetlands triggered by repeated El Niño Southern Oscillation. Glob Change Biol (2017) 23(11):4706–16. doi: 10.1111/gcb.13726
55. Schaefer H, Smale D, Nichol SE, Bromley TM, Brailsford GW, Martin RJ, et al. Limited impact of el Niño–Southern Oscillation on variability and growth rate of atmospheric methane. Biogeosciences (2018) 15(21):6371–86. doi: 10.5194/bg-15-6371-2018
56. McNicol G, Fluet-Chouinard E, Ouyang Z, Knox S, Zhang Z, Aalto T, et al. Upscaling wetland methane emissions from the FLUXNET-CH4 eddy covariance network (UpCH4 v1. 0): model development, network assessment, and budget comparison. AGU Adv (2023) 4(5):e2023AV000956. doi: 10.1029/2023AV000956
57. Shaw JT, Allen G, Barker P, Pitt JR, Pasternak D, Bauguitte SJB, et al. Large methane emission fluxes observed from tropical wetlands in Zambia. Global Biogeochem Cycles (2022) 36(6):e2021GB007261. doi: 10.1029/2021GB007261
58. Pandey S, Houweling S, Lorente A, Borsdorff T, Tsivlidou M, Bloom AA, et al. Using satellite data to identify the methane emission controls of South Sudan’s wetlands. Biogeosciences (2021) 18(2):557–72. doi: 10.5194/bg-18-557-2021
59. Riahi K, Schaeffer R, Arango J, Calvin K, Guivarch C, Hasegawa T, et al. Mitigation pathways compatible with long-term goals. In: Shukla PR, Skea J, Slade R, Al Khourdajie R, van Diemen D, McCollum M, et al, editors. Climate Change 2022: Mitigation of Climate Change. Contribution of Working Group III to the Sixth Assessment Report of the Intergovernmental Panel on Climate Change . Cambridge: Cambridge University Press (2022) 295–408. doi: 10.1017/9781009157926.005
60. Allen MR, Peters GP, Shine KP, Azar C, Balcombe P, Boucher O, et al. Indicate separate contributions of long-lived and short-lived greenhouse gases in emission targets. npj Clim Atmos Sci (2022) 5:5. doi: 10.1038/s41612-021-00226-2
61. Arndt C, Hristov AN, Price WJ, McClelland SC, Pelaez AM, Cueva SF, et al. Full adoption of the most effective strategies to mitigate methane emissions by ruminants can help meet the 1.5°C target by 2030 but not 2050. Proc Natl Acad Sci USA (2022) 119(20):e2111294119. doi: 10.1073/pnas.2111294119
62. Ivanovich CC, Sun T, Gordon DR, Ocko IB. Future warming from global food consumption. Nat Clim Chang (2023) 13(3):297–302. doi: 10.1038/s41558-023-01605-8
63. Forster P, Storelvmo T, Armour K, Collins W, Dufresne J-L, Frame D, et al. The Earth’s energy budget, climate feedbacks, and climate sensitivity. In: Masson-Delmotte V, Zhai AP, Pirani A, Connors SL, Péan C, Berger S, et al, editors. Climate Change 2021: The Physical Science Basis. Contribution of Working Group I to the Sixth Assessment Report of the Intergovernmental Panel on Climate Change . Cambridge: Cambridge University Press (2021) 923–1054. doi: 10.1017/9781009157896.009
64. Collins WJ, Webber CP, Cox PM, Huntingford C, Lowe J, Sitch S, et al. Increased importance of methane reduction for a 1.5 degree target. Environ Res Lett (2018) 13(5):054003. doi: 10.1088/1748-9326/aab89c
65. Rogelj J, Lamboll RD. Substantial reductions in non-CO 2 greenhouse gas emissions reductions implied by IPCC estimates of the remaining carbon budget. Commun Earth Environ (2024) 5:35. doi: 10.1038/s43247-023-01168-8
66. United Nations Environmental Programme. Emissions Gap Report . Nairobi: UNEP (2017). Available at: https://www.unep.org/resources/emissions-gap-report-2017 .
67. Brazzola N, Wohland J, Patt A. Offsetting unabated agricultural emissions with CO 2 removal to achieve ambitious climate targets. PloS One (2021) 16(3):e0247887. doi: 10.1371/journal.pone.0247887
68. Reisinger A, Geden O. Temporary overshoot: origins, prospects, and a long path ahead. One Earth (2023) 6(12):1631–7. doi: 10.1016/j.oneear.2023.11.008
69. Myhre G, Shindell D, Breon F-M, Collins W, Fuglestvedt J, Huang J, et al. Anthropogenic and Natural Radiative Forcing. In: Stocker TF, Qin D, Plattner GK, Tignor M, Allen SK, Boschung J, et al, editors. Climate Change 2013: The Physical Science Basis. Contribution of Working Group I to the Fifth Assessment Report of the Intergovernmental Panel on Climate Change . Cambridge: Cambridge University Press (2013) 659–740. Available at: https://www.cambridge.org/core/books/abs/climate-change-2013-the-physical-science-basis/anthropogenic-and-natural-radiative-forcing/63EB1057C36890FEAA4269F771336D4D .
70. Ocko IB, Hamburg SP, Jacob DJ, Keith DW, Keohane NO, Oppenheimer M, et al. Unmask temporal trade-offs in climate policy debates. Science (2017) 356(6337):492–3. doi: 10.1126/science.aaj2350
71. Shindell D, Borgford-Parnell N, Brauer M, Haines A, Kuylenstierna JCI, Leonard SA, et al. A climate policy pathway for near- and long-term benefits. Science (2017) 356(6337):493–4. doi: 10.1126/science.aak9521
72. Allen MR, Shine KP, Fuglestvedt JS, Millar RJ, Cain M, Frame DJ, et al. A solution to the misrepresentations of CO 2 -equivalent emissions of short- lived climate pollutants under ambitious mitigation. npj Clim Atmos Sci (2018) 1(1):16. doi: 10.1038/s41612-018-0026-8
73. Liu S, Proudman J, Mitloehner FM. Rethinking methane from animal agriculture. J Agric Biol Sci CABI (2021) 2(1):1–13. doi: 10.1186/s43170-021-00041-y
74. Ridoutt B. Climate neutral livestock production–A radiative forcing-based climate footprint approach. J Cleaner Prod (2021) 291:125260. doi: 10.1016/j.jclepro.2020.125260
75. Donnison CL, Murphy-Bokern D. Are climate neutrality claims in the livestock sector too good to be true? Environ Res Lett (2024) 19(1):011001. doi: 10.1088/1748-9326/ad0f75
76. Rogelj J, Schleussner C-F. Unintentional unfairness when applying new greenhouse gas emissions metrics at country level. Environ Res Lett (2019) 14(11):114039. doi: 10.1088/1748-9326/ab4928
77. West JJ, Fiore AM, Horowitz LW. Scenarios of methane emission reductions to 2030: abatement costs and co-benefits to ozone air quality and human mortality. Clim Change (2012) 114(3–4):441–61. doi: 10.1007/s10584-012-0426-4
78. Shindell DT, Fuglestvedt JS, Collins WJ. The social cost of methane: theory and applications. Faraday Discuss (2017) 200:429–51. doi: 10.1039/C7FD00009J
79. Mar KA, Unger C, Walderdorff L, Butler T. Beyond CO 2 equivalence: the impacts of methane on climate, ecosystems, and health. Environ Sci Policy (2022) 134:127–36. doi: 10.1016/j.envsci.2022.03.027
80. Fu B, Gasser T, Li B, Tao S, Ciais P, Piao S, et al. Short-lived climate forcers have long-term climate impacts via the carbon–climate feedback. Nat Clim Change (2020) 10(9):851–5. doi: 10.1038/s41558-020-0841-x
81. Nzotungicimpaye CM, MacIsaac AJ, Zickfeld K. Delaying methane mitigation increases the risk of breaching the 2°C warming limit. Commun Earth Environ (2023) 4(1):250. doi: 10.1038/s43247-023-00898-z
82. Harmsen MJHM, van Vuuren DP, Bodirsky BL, Chateau J, Durand-Lasserve O, Drouet L, et al. The role of methane in future climate strategies: mitigation potentials and climate impacts. Clim Change (2019) 163(3):1409–25. doi: 10.1007/s10584-019-02437-2
83. Howarth RW, Jacobson MZ. How green is blue hydrogen? Energy Sci Eng (2021) 9(10):1676–87. doi: 10.1002/ese3.956
84. Ocko IB, Hamburg SP. Climate consequences of hydrogen emissions. Atmos Chem Phys (2022) 22(14):9349–68. doi: 10.5194/acp-22-9349-2022
85. Bertagni MB, Pacala SW, Paulot F, Porporato A. Risk of the hydrogen economy for atmospheric methane. Nat Commun (2022) 13(1):7706. doi: 10.1038/s41467-022-35419-7
86. Byers E, Krey V, Kriegler E, Riahi K, Schaeffer R, Jarmo K, et al. AR6 Scenarios Database hosted by International Institute for Applied Systems Analysis. (2022). Available at: doi: 10.5281/zenodo.5886911
87. Dooley K, Keith H, Larson A, Catacora-Vargas G, Carton W, Christiansen KL, et al. The Land Gap Report 2022 . (2022). Available at: https://landgap.org/2022/report .
88. Hayek MN, Harwatt H, Ripple WJ, Mueller ND. The carbon opportunity cost of animal-sourced food production on land. Nat Sustain (2021) 4(1):21–4. doi: 10.1038/s41893-020-00603-4
89. Weber J, King JA, Abraham NL, Grosvenor DP, Smith CJ, Shin YM, et al. Chemistry-albedo feedbacks offset up to a third of forestation’s CO 2 removal benefits. Science (2024) 383(6685):860–4. doi: 10.1126/science.adg6196
90. International Energy Agency. Global Methane Tracker 2023 . Paris: IEA (2023). Available at: https://www.iea.org/reports/global-methane-tracker-2023 .
91. Natural Resources Canada. Methane Centre of Excellence (2024). Available at: https://natural-resources.Canada.ca/climate-change/methane-centre-excellence/25656 .
92. Andreae MO, Jones CD, Cox PM. Strong present-day aerosol cooling implies a hot future. Nature (2005) 435(7046):1187–90. doi: 10.1038/nature03671
93. Raes F, Seinfeld JH. New directions: climate change and air pollution abatement: a bumpy road. Atmos Environ (2009) 43(32):5132–3. doi: 10.1016/j.atmosenv.2009.06.001
94. Shindell D, Smith CJ. Climate and air-quality benefits of a realistic phase-out of fossil fuels. Nature (2019) 573(7774):408–11. doi: 10.1038/s41586-019-1554-z
95. Dreyfus GB, Xu Y, Shindell DT, Zaelke D, Ramanathan V. Mitigating Climate Disruption in Time: a self-consistent approach for avoiding both near-term and long-term global warming. Proc Natl Acad Sci USA (2022) 119(22):e2123536119. doi: 10.1073/pnas.2123536119
96. Riahi K, van Vuuren DP, Kriegler E, Edmonds J, O’Neill B, Fujimori S, et al. The Shared Socioeconomic Pathways and their energy, land use, and greenhouse gas emissions implications: an overview. Glob Environ Change (2017) 42:153–68. doi: 10.1016/j.gloenvcha.2016.05.009
97. Staniaszek Z, Griffiths PT, Folberth GA, O’Connor FM, Abraham NL, Archibald AT. The role of future anthropogenic methane emissions in air quality and climate. npj Clim Atmos Sci (2022) 5(1):1–8. doi: 10.1038/s41612-022-00247-5
98. Jones CD, Frölicher TL, Koven C, MacDougall AH, Matthews HD, Zickfeld K, et al. The Zero Emissions Commitment Model Intercomparison Project (ZECMIP) contribution to C4MIP: quantifying committed climate changes following zero carbon emissions. Geosci Model Dev (2019) 12(10):4375–85. doi: 10.5194/gmd-12-4375-2019
99. Palazzo Corner S, Siegert M, Ceppi P, Fox-Kemper B, Frölicher TL, Gallego-Sala A, et al. The Zero Emissions Commitment and climate stabilization. Front Sci (2023) 1:1170744. doi: 10.3389/fsci.2023.1170744
100. Parsons LA, Shindell D, Tigchelaar M, Zhang Y, Spector JT. Increased labor losses and decreased adaptation potential in a warmer world. Nat Commun (2021) 12:7286. doi: 10.1038/s41467-021-27328-y
101. Saunois M, Stavert AR, Poulter B, Bousquet P, Canadell JG, Jackson RB, et al. The global methane budget 2000–2017. Earth Syst Sci Data (2020) 12(3):1561–623. doi: 10.5194/essd-12-1561-2020
102. Nisbet EG, Fisher RE, Lowry D, France JL, Allen G, Bakkaloglu S, et al. Methane mitigation: methods to reduce emissions, on the path to the Paris agreement. Rev Geophys (2020) 58(1):RG000675:e2019. doi: 10.1029/2019RG000675
103. Willett W, Rockström J, Loken B, Springmann M, Lang T, Vermeulen S, et al. Food in the Anthropocene: the EAT–Lancet Commission on healthy diets from sustainable food systems. Lancet (2019) 393(10170):447–92. doi: 10.1016/S0140-6736(18)31788-4
104. Babiker M, Berndes G, Blok K, Cohen B, Cowie A, Geden O, et al. Cross-sectoral perspectives. In: Shukla PR, Skea J, Slade R, Al Khourdajie R, van Diemen D, McCollum M, et al, editors. Climate Change 2022: Mitigation of Climate Change. Contribution of Working Group III to the Sixth Assessment Report of the Intergovernmental Panel on Climate Change . Cambridge: Cambridge University Press (2022) 1245–1354 . Available at: https://www.ipcc.ch/report/ar6/wg3/ .
105. Harrison MT, Cullen BR, Mayberry DE, Cowie AL, Bilotto F, Badgery WB, et al. Carbon myopia: the urgent need for integrated social, economic and environmental action in the livestock sector. Glob Change Biol (2021) 27(22):5726–61. doi: 10.1111/gcb.15816
106. Global Methane Hub and ClimateWorks Foundation. Global Innovation Needs Assessments: Food System Methane [Technical Report] . ClimateWorks Foundation (2023). Available at: https://www.climateworks.org/ginas-methane/ .
107. Wang Y, Guo X, Huo Y, Li M, Pan Y, Yu S, et al. Toward a versatile spaceborne architecture for immediate monitoring of the global methane pledge. Atmos Chem Phys (2023) 23(9):5233–49. doi: 10.5194/acp-23-5233-2023
108. Pandey S, Gautam R, Houweling S, van der Gon HD, Sadavarte P, Borsdorff T, et al. Satellite observations reveal extreme methane leakage from a natural gas well blowout. Proc Natl Acad Sci USA (2019) 116(52):26376–81. doi: 10.1073/pnas.1908712116
109. Varon DJ, Jacob DJ, Jervis D, McKeever J. Quantifying time-averaged methane emissions from individual coal mine vents with GHGSat-D satellite observations. Environ Sci Technol (2020) 54(16):10246–53. doi: 10.1021/acs.est.0c01213
110. Sadavarte P, Pandey S, Maasakkers JD, Lorente A, Borsdorff T, Denier van der Gon H, et al. Methane emissions from superemitting coal mines in Australia quantified using TROPOMI satellite observations. Environ Sci Technol (2021) 55(24):16573–80. doi: 10.1021/acs.est.1c03976
111. de Foy B, Schauer JJ, Lorente A, Borsdorff T. Investigating high methane emissions from urban areas detected by TROPOMI and their association with untreated wastewater. Environ Res Lett (2023) 18(4):044004. doi: 10.1088/1748-9326/acc118
112. Maasakkers JD, Varon DJ, Elfarsdóttir A, McKeever J, Jervis D, Mahapatra G, et al. Using satellites to uncover large methane emissions from landfills. Sci Adv (2022) 8(31):eabn9683. doi: 10.1126/sciadv.abn9683
113. Chen Z, Jacob DJ, Nesser H, Sulprizio MP, Lorente A, Varon DJ, et al. Methane emissions from China: a high-resolution inversion of TROPOMI satellite observations. Atmos Chem Phys (2022) 22(16):10809–26. doi: 10.5194/acp-22-10809-2022
114. United States Environmental Protection Agency. Report on the Social Cost of Greenhouse Gases: Estimates Incorporating Recent Scientific Advances . Washington, DC: U.S. EPA (2023). Available at: https://www.epa.gov/environmental-economics/scghg .
115. Climate Impact Lab. Data-Driven Spatial Climate Impact Model User Manual. [Version 092022-EPA] . Climate Impact Lab (2022). Available at: https://impactlab.org/research/dscim-user-manual-version-092022-epa .
116. Rennert K, Errickson F, Prest BC, Rennels L, Newell RG, Pizer W, et al. Comprehensive evidence implies a higher social cost of CO 2 . Nature (2022) 610(7933):687–92. doi: 10.1038/s41586-022-05224-9
117. Howard PH, Sterner T. Few and not so far between: a meta-analysis of climate damage estimates. Environ Resour Econ (2017) 68(1):197–225. doi: 10.1007/s10640-017-0166-z
118. Wang T, Teng F. Damage function uncertainty increases the social cost of methane and nitrous oxide. Nat Clim Change (2023) 13(11):–1258–65. doi: 10.1038/s41558-023-01803-4
119. Melvin AM, Sarofim MC, Crimmins AR. Climate benefits of U.S. EPA programs and policies that reduced methane emissions 1993–2013. Environ Sci Technol (2016) 50(13):6873–81. doi: 10.1021/acs.est.6b00367
120. Sarofim MC, Waldhoff ST, Anenberg SC. Valuing the ozone-related health benefits of methane emission controls. Environ Resour Econ (2017) 66(1):45–63. doi: 10.1007/s10640-015-9937-6
121. Vandyck T, Keramidas K, Tchung-Ming S, Weitzel M, Van Dingenen R. Quantifying air quality co-benefits of climate policy across sectors and regions. Clim Change (2020) 163(3):1501–17. doi: 10.1007/s10584-020-02685-7
122. Sampedro J, Waldhoff S, Sarofim M, Van Dingenen R. Marginal damage of methane emissions: ozone impacts on agriculture. Environ Resour Econ (2023) 84(4):1095–126. doi: 10.1007/s10640-022-00750-6
123. California Air Resources Board. Cap-and-Trade Program (2023). Available at: https://ww2.arb.ca.gov/our-work/programs/cap-and-trade-program .
124. Parry I, Black S, Minnett D, Mylonas V, Vernon N. How to cut methane emissions. IMF Staff Climate Note 2022/008 . Washington, DC: International Monetary Fund (2022).
125. Nordhaus W. Projections and uncertainties about climate change in an era of minimal climate policies. Am Econ J Econ Policy (2018) 10(3):333–60. doi: 10.1257/pol.20170046
126. International Energy Agency. Methane emissions remained stubbornly high in 2022 even as soaring energy prices made actions to reduce them cheaper than ever (2023). Available at: https://www.iea.org/news/methane-emissions-remained-stubbornly-high-in-2022-even-as-soaring-energy-prices-made-actions-to-reduce-them-cheaper-than-ever .
127. International Energy Agency. Global Methane Tracker 2024 . Paris: IEA (2024). Available at: https://www.iea.org/reports/global-methane-tracker-2024 .
128. Buonocore J, Reka S, Yang D, Chang C, Roy A, Thompson T, et al. Air pollution and health impacts of oil & gas production in the United States. Env Res Health (2023) 1(2):021006. doi: 10.1088/2752-5309/acc886
129. Chen C, McCabe DC, Fleischman LE, Cohan DS. Black carbon emissions and associated health impacts of gas flaring in the United States. Atmosphere (2022) 13(3):385. doi: 10.3390/atmos13030385
130. Emanuel RE, Caretta MA, Rivers IIIL, Vasudevan P. Natural gas gathering and transmission pipelines and social vulnerability in the United States. GeoHealth (2021) 5(6):e2021GH000442. doi: 10.1029/2021GH000442
131. O’Connor FM, Johnson BT, Jamil O, Andrews T, Mulcahy JP, Manners J. Apportionment of the pre-industrial to present-day climate forcing by methane using UKESM1: the role of the cloud radiative effect. J Adv Model Earth Syst (2022) 14(10):e2022MS002991. doi: 10.1029/2022MS002991
132. Smith CJ, Kramer RJ, Myhre G, Forster PM, Soden BJ, Andrews T, et al. Understanding rapid adjustments to diverse forcing agents. Geophys Res Lett (2018) 45(21):12–023. doi: 10.1029/2018GL079826
133. Allen RJ, Zhao X, Randles CA, Kramer RJ, Samset BH, Smith CJ. Surface warming and wetting due to methane’s long-wave radiative effects muted by short-wave absorption. Nat Geosci (2023) 16(4):314–20. doi: 10.1038/s41561-023-01144-z
134. Jackson RB, Abernethy S, Canadell JG, Cargnello M, Davis SJ, Féron S, et al. Atmospheric methane removal: a research agenda. Philos Trans A Math Phys Eng Sci (2021) 379(2210):20200454. doi: 10.1098/rsta.2020.0454
135. International Energy Agency. World Energy Outlook 2018 . Paris: IEA (2018). Available at: https://www.iea.org/reports/world-energy-outlook-2018 .
136. Alexandratos N, Bruisma J. World Agriculture Towards 2030/2050–The 2012 Revision, ESA Working Paper No. 12–03 . Rome: Agricultural Development Economics Unit, Food and Agricultural Organization of the United Nations (2012). Available at: https://www.fao.org/4/ap106e/ap106e.pdf .
137. Robinson S, Mason d’Croz D, Islam S, Sulser TB, Robertson RD, Zhu T, et al. The International Model for Policy Analysis of Agricultural Commodities and Trade (Impact): model description for version 3 [IFRI discussion paper 1483]. Washington, DC: International Food Policy Research Institute (2015). Available at: https://www.ifpri.org/publication/international-model-policy-analysis-agricultural-commodities-and-trade-impact-model-0 .
138. Bernal B, Murray LT, Pearson TRH. Global carbon dioxide removal rates from forest landscape restoration activities. Carbon Balance Manag (2018) 13(1):22. doi: 10.1186/s13021-018-0110-8
139. Geoffroy O, Saint-Martin D, Bellon G, Voldoire A, Olivié DJL, Tytéca S. Transient climate response in a two-layer energy-balance model. Part II: Representation of the efficacy of deep-ocean heat uptake and validation for CMIP5 AOGCMs. J Climate (2013) 26(6):1859–76. doi: 10.1175/JCLI-D-12-00196.1
140. Turner MC, Jerrett M, Pope CA, Krewski D, Gapstur SM, Diver WR, et al. Long-term ozone exposure and mortality in a large prospective study. Am J Respir Crit Care Med (2016) 193(10):1134–42. doi: 10.1164/rccm.201508-1633OC
141. Lim CC, Hayes RB, Ahn J, Shao Y, Silverman DT, Jones RR, et al. Long-term exposure to ozone and cause-specific mortality risk in the United States. Am J Respir Crit Care Med (2019) 200(8):1022–31. doi: 10.1164/rccm.201806-1161OC
142. United States Environmental Protection Agency. Integrated Science Assessment (ISA) for Ozone and Related Photochemical Oxidants (Final report, Apr 2020) . Washington, DC: U.S. EPA (2020). Available at: https://cfpub.epa.gov/ncea/isa/recordisplay.cfm?deid=348522 .
143. Niu Y, Zhou Y, Chen R, Yin P, Meng X, Wang W, et al. Long-term exposure to ozone and cardiovascular mortality in China: a nationwide cohort study. Lancet Planet Health (2022) 6(6):e496–503. doi: 10.1016/S2542-5196(22)00093-6
144. Liu S, Zhang Y, Ma R, Liu X, Liang J, Lin H, et al. Long-term exposure to ozone and cardiovascular mortality in a large Chinese cohort. Environ Int (2022) 165:107280. doi: 10.1016/j.envint.2022.107280
145. Xue T, Wang R, Tong M, Kelly FJ, Liu H, Li J, et al. Estimating the exposure–response function between long-term ozone exposure and under-5 mortality in 55 low-income and middle-income countries: a retrospective, multicentre, epidemiological study. Lancet Planet Health (2023) 7(9):e736–46. doi: 10.1016/S2542-5196(23)00165-1
146. Center for International Earth Science Information Network, Columbia University, Food and Agriculture Programme of the United Nations, and Centro Internacional de Agricultura Tropical. Gridded Population of the World, Version 4 (GPWv4): Population Count Grid . Palisades, NY: National Aeronautics and Space Administration Socioeconomic Data and Applications Center (2016). Available at: https://sedac.ciesin.columbia.edu/data/collection/gpw-v4 .
147. Global Burden of Disease Collaborative Network. Global Burden of Disease Study 2017 (GBD 2017) . Seattle, WA: The Institute for Health Metrics and Evaluation (2017).
148. Kjellstrom T, Freyberg C, Lemke B, Otto M, Briggs D. Estimating population heat exposure and impacts on working people in conjunction with climate change. Int J Biometeorol (2018) 62(3):291–306. doi: 10.1007/s00484-017-1407-0
149. Foster J, Smallcombe JW, Hodder S, Jay O, Flouris AD, Nybo L, et al. An advanced empirical model for quantifying the impact of heat and climate change on human physical work capacity. Int J Biometeorol (2021) 65(7):1215–29. doi: 10.1007/s00484-021-02105-0
150. International Organization for Standardization. Ergonomics of the Thermal Environment — Assessment of Heat Stress Using the WBGT (Wet Bulb Globe Temperature) index [ISO Standard 7243:2017] . Geneva: ISO (2017). Available at: https://www.iso.org/standard/67188.html .
151. Bröde P, Fiala D, Lemke B, Kjellstrom T. Estimated work ability in warm outdoor environments depends on the chosen heat stress assessment metric. Int J Biometeorol (2018) 62(3):331–45. doi: 10.1007/s00484-017-1346-9
152. International Labour Organization. Employment by sex and age — ILO modelled estimates. ILOSTAT Database (2023). Available at: https://ilostat.ilo.org/data .
153. World Bank. World Bank Open Data (2021). Available at: https://data.worldbank.org/ .
Keywords: methane emissions, climate change mitigation, ozone, CO 2 budget, mitigation costs, fossil fuels, net zero, livestock
Citation: Shindell D, Sadavarte P, Aben I, Bredariol TdO, Dreyfus G, Höglund-Isaksson L, Poulter B, Saunois M, Schmidt GA, Szopa S, Rentz K, Parsons L, Qu Z, Faluvegi G and Maasakkers JD. The methane imperative. Front Sci (2024) 2:1349770. doi: 10.3389/fsci.2024.1349770
Received: 05 December 2023; Accepted: 06 June 2024; Published: 30 July 2024.
Reviewed by:
Copyright © 2024 Shindell, Sadavarte, Aben, Bredariol, Dreyfus, Höglund-Isaksson, Poulter, Saunois, Schmidt, Szopa, Rentz, Parsons, Qu, Faluvegi and Maasakkers. This is an open-access article distributed under the terms of the Creative Commons Attribution License (CC BY) . The use, distribution or reproduction in other forums is permitted, provided the original author(s) and the copyright owner(s) are credited and that the original publication in this journal is cited, in accordance with accepted academic practice. No use, distribution or reproduction is permitted which does not comply with these terms.
*Correspondence: Drew Shindell, [email protected]
Disclaimer: All claims expressed in this article are solely those of the authors and do not necessarily represent those of their affiliated organizations, or those of the publisher, the editors and the reviewers. Any product that may be evaluated in this article or claim that may be made by its manufacturer is not guaranteed or endorsed by the publisher.
Follow the science, follow Frontiers in Science
Apple Intelligence Foundation Language Models
View publication
Copy Bibtex
We present foundation language models developed to power Apple Intelligence features, including a ∼3 billion parameter model designed to run efficiently on devices and a large server-based language model designed for Private Cloud Compute. These models are designed to perform a wide range of tasks efficiently, accurately, and responsibly. This report describes the model architecture, the data used to train the model, the training process, how the models are optimized for inference, and the evaluation results. We highlight our focus on Responsible AI and how the principles are applied throughout the model development.
This paper provides technical details for Apple’s On-Device and Server Foundation Models, introduced on June 10, 2024, in this post .
Related readings and updates.
Introducing apple’s on-device and server foundation models.
At the 2024 Worldwide Developers Conference , we introduced Apple Intelligence, a personal intelligence system integrated deeply into iOS 18, iPadOS 18, and macOS Sequoia.
Apple Intelligence is comprised of multiple highly-capable generative models that are specialized for our users’ everyday tasks, and can adapt on the fly for their current activity. The foundation models built into Apple Intelligence have been fine-tuned for user experiences such as writing and refining text, prioritizing and summarizing notifications, creating playful images for conversations with family and friends, and taking in-app actions to simplify interactions across apps.
Apple Natural Language Understanding Workshop 2023
Earlier this year, Apple hosted the Natural Language Understanding workshop. This two-day hybrid event brought together Apple and members of the academic research community for talks and discussions on the state of the art in natural language understanding.
In this post, we share highlights from workshop discussions and recordings of select workshop talks.
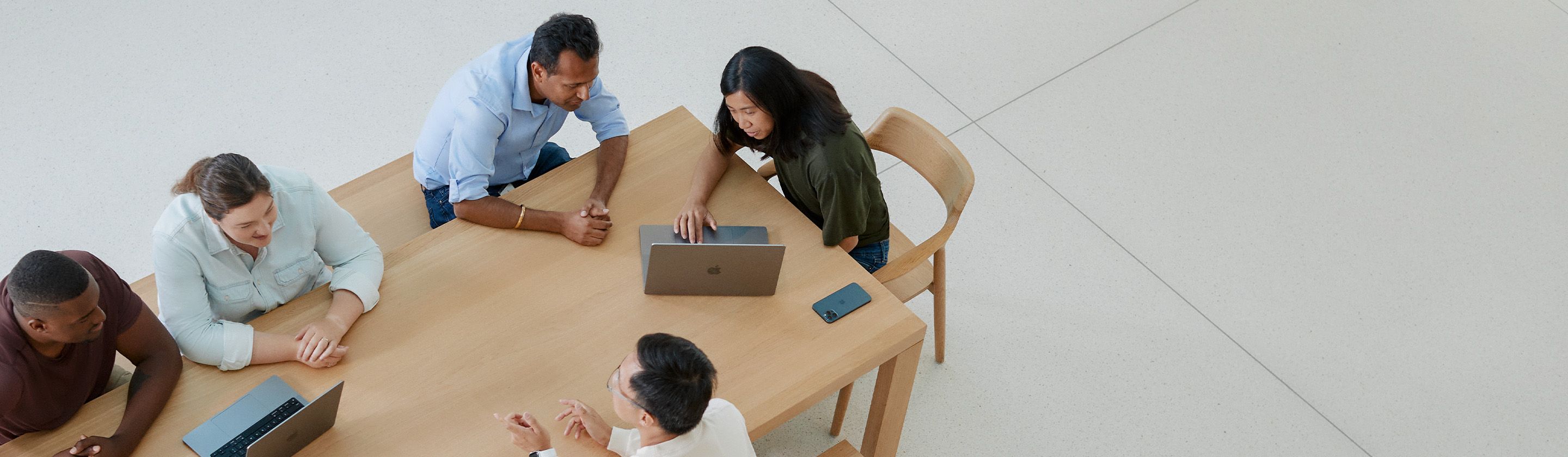
Discover opportunities in Machine Learning.
Our research in machine learning breaks new ground every day.
Work with us
- Featured Paper of the Month
- Reviews to Read
- Hot off the Press
- Technology Development Initiative Paper of the Month
- Seminar Series
- Addiction Grand Rounds
- About NIDA IRP
- Directions and Map
- Careers at NIDA IRP
- Emergency Contacts
- Office of the Scientific Director
- Office of the Clinical Director
- Office of Education and Career Development
- Administrative Management Branch
- Molecular Targets and Medications Discovery Branch
- Cellular and Neurocomputational Systems Branch
- Molecular Neuropsychiatry Research Branch
- Neuroimaging Research Branch
- Behavioral Neuroscience Research Branch
- Integrative Neuroscience Research Branch
- Translational Addiction Medicine Branch
- Core Facilities
- Women Scientist Advisors
- Community Outreach Group
- OECD Awards
- Summer Internship Program
- Postbaccalaureate Program
- Graduate Partnership Program
- Postdoctoral Program
- Diversity, Equity, Inclusion and Access Initiatives (DEIA)
- NIDA Speakers Bureau
- Clinical Electives Program
- Study Volunteers
- Skip to primary navigation
- Skip to main content
- Skip to primary sidebar
National Institute on Drug Abuse - Intramural Research Program
- TDI Paper of the Month
- TDI Seminar Series
- TDI Paper of the Month Committee
- Technology Transfer
- Transgenic Rat Project
- Equipment Inventory Database (NIDA Staff Only, VPN Required)
Technology Development Initiative – Paper of the Month – August 2024
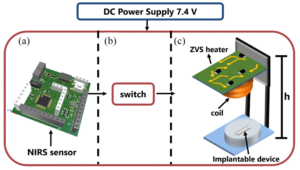
A figure from this article. Image copyright IEEE
A Wearable Device Towards Automatic Detection and Treatment of Opioid Overdose
Published in IEEE Transactions on Biomedical Circuits and Systems .
Juan C Mesa, Michael D MacLean, Maria Ms, Alan Nguyen, Rujuta Patel, Timothy Diemer, Jongcheon Lim, Chi Hwan Lee, Hyowon Lee
Paper presented by Dr. Agnieszka Sulima and selected by the NIDA TDI Paper of the Month Committee
Publication Brief Description
Opioid use disorder (OUD) and fatal overdose due to intentional and unintentional consumption of opioids, particularly fentanyl and fentanyl-laced mixtures, are emerging global concerns. According to CDC estimates, more than 81,000 deaths were attributed to opioids in 2022 alone. Accessibility to naloxone as a harm reduction intervention for people at risk for opioid overdose is critical in combating opioid-related deaths. Researchers at Purdue University demonstrated the feasibility of a minimally invasive automatic naloxone delivery system that can administer a dose of naloxone upon detection of overdose-induced respiratory failure. The system consists of (1) a near-infrared spectroscopy (NIRS) sensor for detecting hypoxia event, (2) a MOSFET switch driver to integrate the NIRS circuit to a zero-voltage switching (ZVS) driver, (3) a ZVS electromagnetic filed generator to activate a drug delivery device in the presence of hypoxia event, and (4) an implantable drug delivery capsule. It is the first wearable closed-loop device utilizing NIRS sensor to detect and treat an overdose event. Furthermore, the researchers demonstrated that the device is capable of a rapid, within 10 seconds, delivery of a dose of naloxone after detecting a hypoxic event. Although further studies and optimizations are needed, the proof-of-concept closed-loop naloxone delivery system has the potential to reduce opioid toxicity in the absence of bystanders.
Mesa, Juan C; MacLean, Michael D; Ms, Maria; Nguyen, Alan; Patel, Rujuta; Diemer, Timothy; Lim, Jongcheon; Lee, Chi Hwan; Lee, Hyowon
A Wearable Device Towards Automatic Detection and Treatment of Opioid Overdose Journal Article
In: IEEE Trans Biomed Circuits Syst, vol. 18, no. 2, pp. 396–407, 2024 , ISSN: 1940-9990 .
Abstract | Links
- https://pubmed.ncbi.nlm.nih.gov/37938943/
- doi:10.1109/TBCAS.2023.3331272

- National Institute on Drug Abuse
- NIH Intramural Research Program
- National Institutes of Health
- Health and Human Services
- Treatment Information
- Accessibility
- HHS Vulnerability Disclosure
- Freedom of Information Act
- Document Viewing Tools
- Offsite Links
Apple used Google's chips to train two AI models, research paper shows
- Medium Text

Sign up here.
Reporting by Max A. Cherney in San Francisco; Editing by Matthew Lewis and Varun H K
Our Standards: The Thomson Reuters Trust Principles. , opens new tab
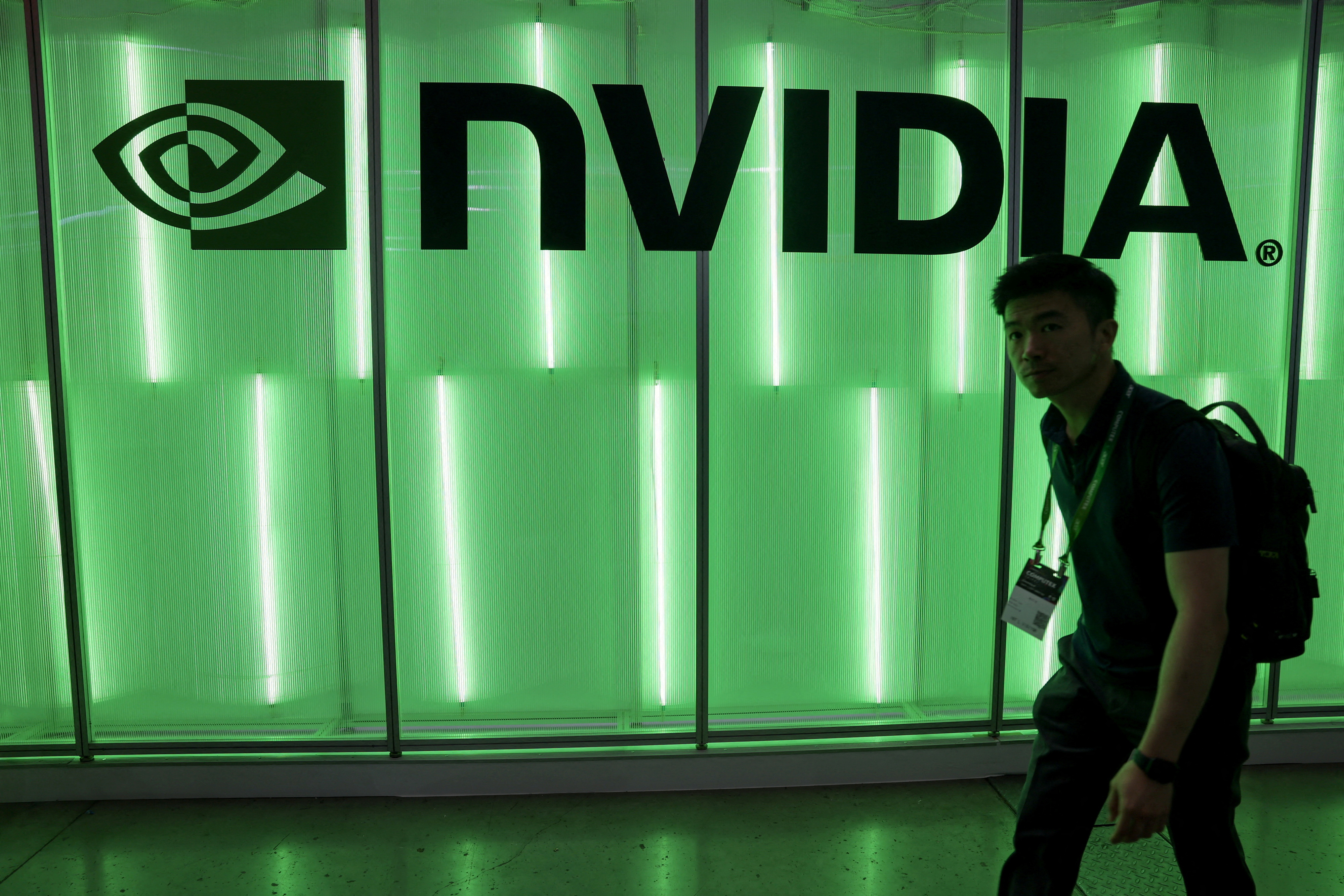
Nvidia shares fall 2% in Frankfurt after media report of US probe
Nvidia shares fell 2.3% in Frankfurt on Friday, following media reports of the U.S. government launching an antitrust probe into the company following complaints from rival chipmakers, and alongside a broad sell off in tech stocks.
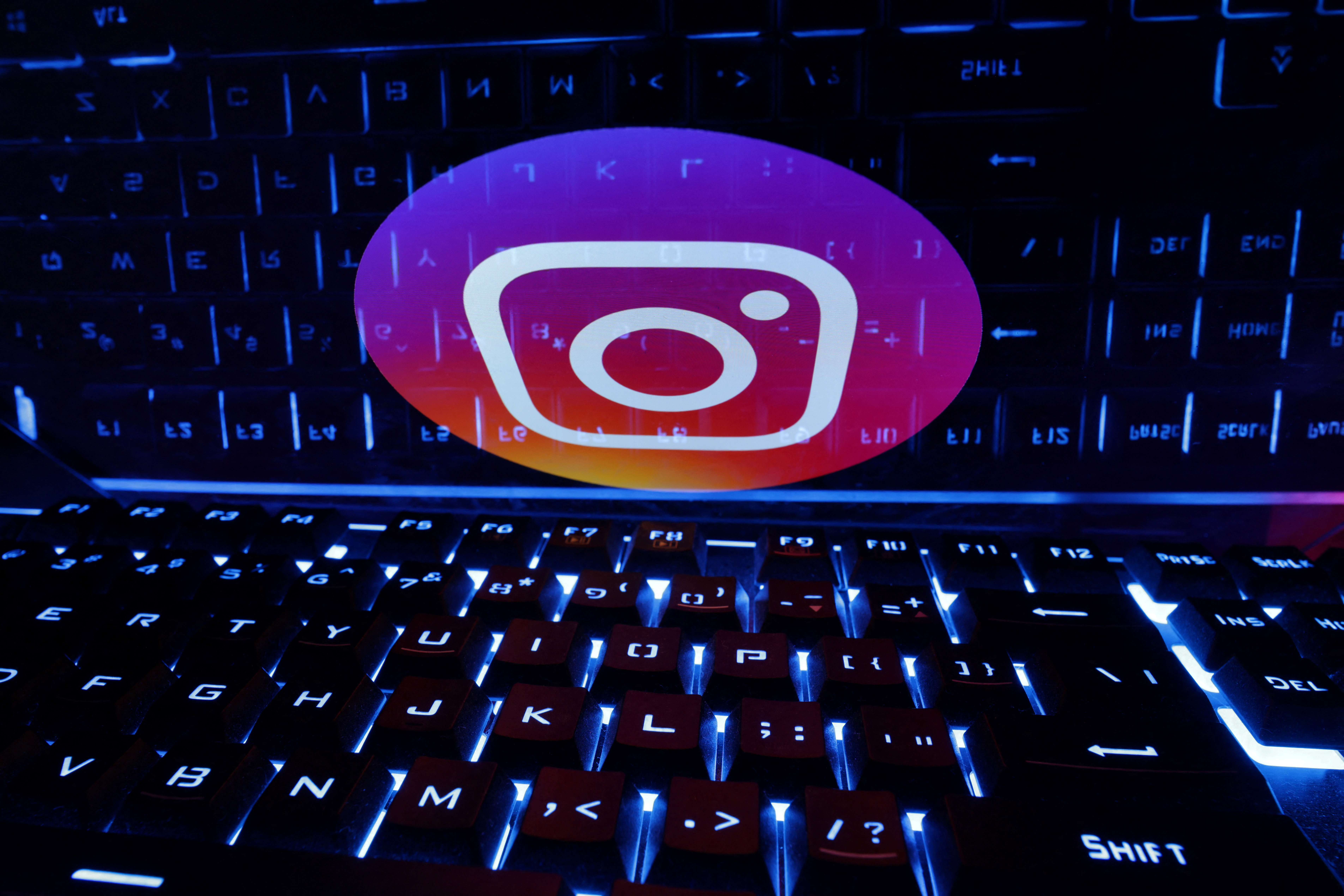

IMAGES
VIDEO
COMMENTS
Google Scholar provides a simple way to broadly search for scholarly literature. Search across a wide variety of disciplines and sources: articles, theses, books, abstracts and court opinions.
Find the research you need | With 160+ million publications, 1+ million questions, and 25+ million researchers, this is where everyone can access science
Promote your research: Once your paper is published, promote it on social media and other online platforms. This will increase the visibility of your work and help it reach a wider audience. Journals and Conferences for Free Research Paper publications. Here are the websites of the open-access journals and conferences mentioned: Open-Access ...
Get 30 days free. 1. Google Scholar. Google Scholar is the clear number one when it comes to academic search engines. It's the power of Google searches applied to research papers and patents. It not only lets you find research papers for all academic disciplines for free but also often provides links to full-text PDF files.
Organize your papers in one place. Try Paperpile. 1. Scopus. Scopus is one of the two big commercial, bibliographic databases that cover scholarly literature from almost any discipline. Besides searching for research articles, Scopus also provides academic journal rankings, author profiles, and an h-index calculator. 2.
These communities are active participants in research and authorship, conferences, and important conversations about today's most relevant technical topics locally and globally. ... which are recognized by academia and industry worldwide as the most vital collection of consolidated published papers in electrical engineering, computer science ...
Access 160+ million publications and connect with 25+ million researchers. Join for free and gain visibility by uploading your research.
Finding recent papers. Your search results are normally sorted by relevance, not by date. To find newer articles, try the following options in the left sidebar: click "Since Year" to show only recently published papers, sorted by relevance; click "Sort by date" to show just the new additions, sorted by date;
Communicating research findings is an essential step in the research process. Often, peer-reviewed journals are the forum for such communication, yet many researchers are never taught how to write a publishable scientific paper. In this article, we explain the basic structure of a scientific paper and describe the information that should be included in each section. We also identify common ...
Free access to millions of research papers for everyone. OA.mg is a search engine for academic papers. Whether you are looking for a specific paper, or for research from a field, or all of an author's works - OA.mg is the place to find it. Universities and researchers funded by the public publish their research in papers, but where do we ...
Advanced. Journal List. PubMed Central ® (PMC) is a free full-text archive of biomedical and life sciences journal literature at the U.S. National Institutes of Health's National Library of Medicine (NIH/NLM)
Journal Top 100 - 2022. This collection highlights our most downloaded* research papers published in 2022. Featuring authors from around the world, these papers highlight valuable research from an ...
Journal Top 100. This collection highlights our most downloaded* research papers published in 2020. Featuring authors from around the world, these papers showcase valuable research from an ...
The introduction section should be approximately three to five paragraphs in length. Look at examples from your target journal to decide the appropriate length. This section should include the elements shown in Fig. 1. Begin with a general context, narrowing to the specific focus of the paper.
research articles. Research articles. Filter By: Article Type. All. All; Article (205779) Conference Proceeding (56) Matters Arising (53) ... Calls for Papers Editor's Choice ...
3. Submit your article according to the journal's submission guidelines. Go to the "author's guide" (or similar) on the journal's website to review its submission requirements. Once you are satisfied that your paper meets all of the guidelines, submit the paper through the appropriate channels.
For example, some journals have a longer history, broader focus and higher impact factor, a measure of how often papers in the journal are cited compared to how much is published in the journal. For more narrowly focused research, there are journals focused on subdisciplines that are well-respected by experts and have high impact factors.
Research articles. Filter By: Article Type. All. All; Appointments Vacant (974) Article (23444) Brief Communication (1079) Brief Communications Arising (580) British Association Supplement (88)
ScienceOpen is a research, networking, and discovery platform. We specialize in: Smart search and discovery within an interactive interface. Researcher promotion and ORCID integration. Open evaluation with article reviews and Collections. Business model based on providing services to publishers.
The journals Nature and Science are where some of the highest quality research is showcased to the world.. The institutions listed below were the largest contributors to papers published in Nature ...
The predicted/observed mean Tropical Cyclone Rainrate (TCR) within 600 km of the TC center (R < 600): for (A) the non-DOD model and (B) the DOD model using the scatter density plot (out-of-sample predictions are made for five testing sets and then combined, then 100 bins with equal intervals are generated for the TCR ranges.The count of scatters is summarized within each box); (C) difference ...
Explore the latest full-text research PDFs, articles, conference papers, preprints and more on RESEARCH PAPERS. Find methods information, sources, references or conduct a literature review on ...
Education. April 27, 2022. Millions of research papers are published in a year. How do scientists keep up? by Eva Botkin-Kowacki, Northeastern University. If you want to be a scientist, you're ...
arXiv.org
The SBTi releases research on considerations for a more effective approach to scope 3 emissions. This is an early step in the review of the Corporate Net-Zero Standard; guidance remains unchanged until process is complete. ... Scope 3 discussion paper: ... This is the first of the three distinct reports that will be published as outlined in the ...
The atmospheric methane growth rates of the 2020s far exceed the latest baseline projections; methane emissions need to drop rapidly (as do CO 2 emissions) to limit global warming to 1.5°C or 2°C.; The abrupt and rapid increase in methane growth rates in the early 2020s is likely attributable largely to the response of wetlands to warming with additional contributions from fossil fuel use ...
content type paper | published July 2024. ... This paper provides technical details for Apple's On-Device and Server Foundation Models, introduced on June 10, 2024, in this post. ... Our research in machine learning breaks new ground every day. Work with us. Machine Learning Research;
The National Institute on Drug Abuse Intramural Research Program is a research facility in Baltimore, MD. ... Published in IEEE Transactions on Biomedical Circuits and Systems ... Alan Nguyen, Rujuta Patel, Timothy Diemer, Jongcheon Lim, Chi Hwan Lee, Hyowon Lee. Paper presented by Dr. Agnieszka Sulima and selected by the NIDA TDI Paper of the ...
Today, we are releasing the team's first paper, which introduces a new research direction for empirically aligning superhuman models. Current alignment methods, such as reinforcement learning from human feedback (RLHF), rely on human supervision. However, future AI systems will be capable of extremely complex and creative behaviors that will ...
Apple relied on chips designed by Google rather than industry leader Nvidia to build two key components of its artificial intelligence software infrastructure for its forthcoming suite of AI tools ...